Dr Graham Exelby May 2024
This paper is part 2 of a series looking at the immune dysfunction, causes of cognitive impairment and other problems, and management protocols.
There are a number of ways that Covid can cause cognitive impairment. Severe Covid can be equivalent to 20 years of aging, with studies showing reduced mental capacity up to 2 years after infection. It is worth noting that no such deficits were seen in patients who had full recovery from Covid.(1) Multiple factors thought to contribute to neurological dysfunction in Long-COVID. These include persistent systemic inflammation with cytokine production, immune system activation and production of Reactive Oxygen Species (ROS).
Each patient requires a careful assessment and likely evaluation of the involved pathology. Cognitive impairment is a major symptom in POTS and its co-morbidities, and the combination of autonomic dysfunction, mechanical “drivers” all can contribute to cognitive impairment and other post-COVID symptoms, thus providing the potential for improvements based on these “drivers.”
The underlying causes may be elucidated by looking at previous history and co-morbidities as it can uncover areas where other treatment can be commenced, eg an underlying mechanical causes eg Thoracic Outlet Syndrome or Nutcracker Syndrome, as well as diet, and the multitude of environmental factors such as mould which are detailed in the series of POTS articles.
Introduction: The Infection
SARS‐CoV‐2 enters the body and its spike (S) protein interacts with ACE2 receptors to infect respiratory epithelial and immune cells. During infection, rapid viral replication causes a strong immune response and immune dysregulation.(2)
At the early stage, the virus infects the lung epithelial cells and is slowly transmitted to the other organs including the gastrointestinal tract, blood vessels, kidneys, heart, and brain. The neurological effect of the virus is mainly due to hypoxia‐driven reactive oxygen species (ROS) and generated cytokine storm. Internalization of SARS‐CoV‐2 triggers ROS production and modulation of the immunological cascade which ultimately initiates the hypercoagulable state and vascular thrombosis. (3) Immune cells are extensively activated and secrete large amounts of inflammatory factors, causing excessive inflammation and the “cytokine storm,” which can lead to immunopathological impairment of COVID‐19, closely related to the severity of the disease. (2)
The hypercoagulability with alterations in haemostatic markers including high D-dimer levels, together with findings of fibrin-rich microthrombi, widespread extracellular fibrin deposition in affected various organs and hypercytokinemia, reveals COVID-19 to be a thrombo-inflammatory disease. Endothelial cells that constitute the lining of blood vessels are the primary targets of a thrombo-inflammatory response, and being highly heterogeneous in their structure and function, differences in the endothelial cells may govern the susceptibility of organs to COVID-19.(4)
Sashindranath and Nandurkar (4) described an established link between COVID-19 and neurological symptoms in up to 50% of patients, including loss of smell and taste, necrotizing encephalitis, seizures, and rarely, Guillain-Barre syndrome. Acute cerebrovascular disease with a relatively low mean age (ranging from 45 to 67 years) is a significant complication of COVID-19. “That the overall incidence of large vessel occlusions is 2-fold higher than in normal acute ischaemic stroke cases and they occur among patients from all age groups, even those without risk factors or comorbidities, strongly implicates COVID-19-related hypercoagulability as the underlying cause. The endothelium has a pivotal role in cerebrovascular disease. Endothelial dysfunction occurs after stroke and leads to oxidative stress, inflammation, increased vascular tone, blood-brain barrier (BBB) damage, and further thrombovascular complications in the brain.”(4)
The pathophysiology of COVID-19 is characterized by systemic inflammation, hypoxia resulting from respiratory failure, and neuroinflammation (either due to viral neurotropism, the ability of the virus to invade and live in neural tissue, or in response to the cytokine storm), all affecting the brain. (5)
The brain and spinal cord, which make up the CNS, are not usually accessed directly by pathogenic factors in the body's circulation due to a series of endothelial cells (single layer of squamous cells that form an interface between circulating blood or lymph in the vessels, controlling the flow of substances into and out of a tissue) known as the blood -brain barrier (BBB) (formed from endothelial cells, astrocyte end-feet and pericytes.)
The BBB prevents most infections from reaching the vulnerable nervous tissue. In the case where infectious agents are directly introduced to the brain or cross the blood–brain barrier, microglial cells must react quickly to decrease inflammation and destroy the infectious agents before they damage the sensitive neural tissue, so basically are the “first responders” in infections.
In humans there are 10 types of body threat receptors, or Toll-Like Receptors (TLRs) that respond to a variety of PAMPs (pathogen-associated molecular patterns associated with bacteria and viruses). TLRs are crucial components in the initiation of the innate immune system, triggering the downstream production of pro-inflammatory cytokines, interferons (IFNs) and other mediators. TLRs recognize invading pathogens by sensing PAMP and activate the regulation of innate immunity and cytokines. TLR activation leads to the production of proinflammatory cytokines and Interferon IFN.(6)
TLRs 1,2,4,5,6,10 are plasma protein TLRs, while TLR3 and 7 are on endosomes (intracellular sorting organelles). TLR2/6 and TLR4 are located on the cell membrane.
TLR2 senses the SARS-CoV-2 envelope protein (E), resulting in production of inflammatory cytokines and chemokines, contributing to the hyperinflammatory state and tissue damage seen in severe Covid.(78)(82) The severity of the Covid infection is largely determined by the E Protein /TLR2 activation rather than the S protein.(6)(7)
TLR4 signalling is activated by the Spike protein (S). This can lead to a pro-thrombotic and pro-inflammatory state contributing to severe complications eg myocardial infarction and acute lung injury.(8)(9)
The endosomal TLR3 senses intracellular viral dsRNA. Activated TLR regulates the production of proinflammatory factors through a series of signalling in the NF‐κB pathway and activates IRF3/7 to produce I IFN. (6)(10) A DNA variant in TLR3 has also been identified as increasing susceptibility and mortality to acute COVID infections by decreasing TLR3 expression and impairing recognition of SARS-Co-V dsRNA. (6) These results suggest perivascular inflammation may be a critical factor in Long COVID, but the role of these receptors in Long COVID associated POTS has not been established.
Damage to the brain triggers a specific type of reactive response mounted by neuroglia cells, in particular by microglia, the most prominent immune cells in the CNS and which are the first to respond to threat.(5) Inflammatory microglial activation (IL-6 and TNFa) is the most common brain pathology found in patients who died of COVID-19: 42% are affected, and another 15% have microclots in brain tissue.(11)
This is complicated by astrocyte/ microglial “cross-talk” and neurotransmitter dysregulation. The SARS-Co-V spike protein activates microglia leading to pro-inflammatory effects and microglial-mediated synapse elimination. This microglial activation and neuroinflammation can disrupt the BBB.
Covid also reduces the morphology and distribution of microglia and astrocytes in the hippocampus which has a major role in learning and memory. Mast cells promote cross-talk between T cells and myeloid cells like microglia during neuroinflammation, and the complex interplay between the activated microglia, reactive astrocytes and mast cells is a key part of the neurological manifestations of the COVID-19 infection.(13)(14)(15)
SARS-CoV-2 preferentially infects and replicates and propagates in astrocytes, particularly those adjacent to infected vasculature. In contrast, neurons and microglia are less likely to be directly infected. Importantly, while microglia and astrocytes are both reactivated, a direct dosage-sensitive effect of SARS-CoV-2 is only observed in reactive astrocytes. Astrocytes are the primary targets of SARS-CoV-2 in the brain. SARS-Co-V preferentially infects astrocytes over neurons resulting in astrocyte reactivation and neuronal death (11).
Figure 1: Mast Cell, Microglia and Astrocyte Cross-Talk
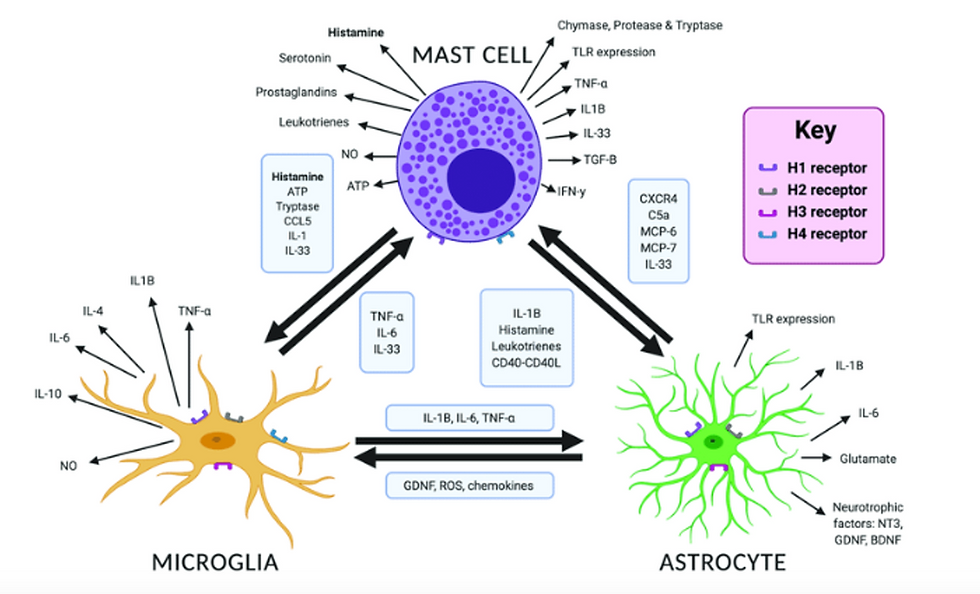
Source: Carthy, Elliott & Ellender, Tommas. (2021). Histamine, Neuroinflammation and Neurodevelopment: A Review. Frontiers in Neuroscience. 15. 10.3389/fnins.2021.680214.(12)
The hypercoagulable state, increased embolic events, microangiopathic thrombosis, and endotheliopathy are all part of the increased stroke (and microclot) risk in SARS‐CoV‐2 infected patients. Viral infections in the cerebrovascular endothelium cause vasculitis in the brain. Thrombotic microangiopathy occurs due to local damage in the cerebrovascular system. ACE2 receptor homeostasis disruption in the cerebrovascular system alters angiotensin level, which also impairs cerebral autoregulation.(4)
Figure 2: Mechanisms involved in neurological complications of COVID-19.
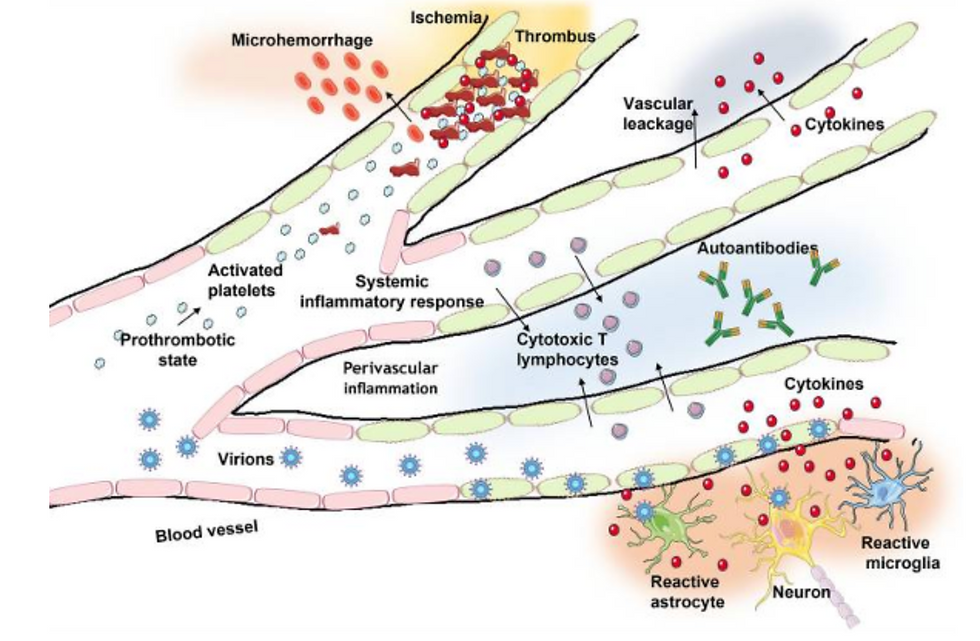
Source: Steardo L Jr, Steardo L, Scuderi C. Astrocytes and the Psychiatric Sequelae of COVID-19: What We Learned from the Pandemic. (11)
Not all emboli and microemboli are venous, as some are arterial, and in some there is bleeding. Mukherjee et al (16) found these arterial emboli are a result of histamine receptor 1 (H1) induced fibrinogen. Rauchet al (17) found phosphatidylserine was associated with increased thrombo-inflammation and vascular complications. The DNA mutations found by Dr Valerio Vittone have contributed significantly to understanding the involved pathways, particularly in patients with DNA mutations eg PEMT, where there are no available biomarkers and may be required to unlock the various mechanisms that are occurring.(18)
Gut Microbiota
In addition to its metabolic role, the gut microbiota also has a key role in the development and regulation of the immune system. Gut microbes coordinate immune homeostasis by producing metabolites, inducing proinflammatory responses and cytokines. The gut microbiota crosstalk with the lung and the gut microbiota affects immune functions in the respiratory epithelium, including the secretion of type I Interferons (IFN‐α and IFN‐β) and cytokines (IL‐10, TGF‐β, and IL22) to limit viral replication.
Natale et al (20) describe that a “large body of evidence shows how gastrointestinal pathologies can affect the CNS bypassing or altering blood-brain barrier (BBB) and related pathways, including the glymphatic system. Furthermore, “via the microbiota-gut-brain axis, triggering Receptors Expressed on Myeloid cells (TREM)-positive activated macrophages along with inflammatory mediators may reach the brain through blood, glymphatic system, circumventricular organs, or the vagus nerve. This may foster pro-inflammatory reactions in the brain, bridging inflammatory bowel disease and neurological disorders.” This is thought to occur from the SARS-CoV-2 viral infection.
Increased blood-brain (BBB) permeability allows cytokines and virus to penetrate into the brain causing neuroinflammation and neuronal and astrocyte damage. Tissue hypoxia may occur from microclot formation, complicated by addition of amyloid to the fibrin clots. (21)(22)(23)
Figure 3: Proposed Mechanisms underlying Neurological Symptoms
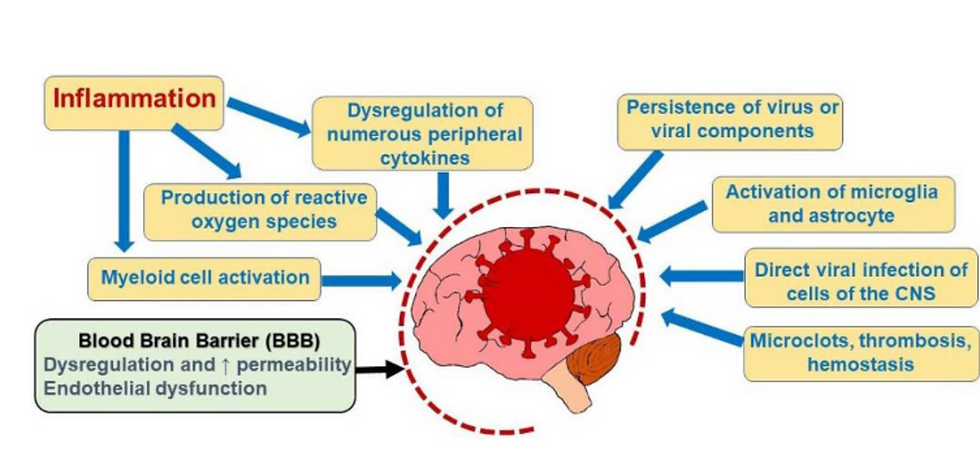
Source: Reiss AB, Greene C, Dayaramani C, Rauchman SH, Stecker MM, De Leon J, Pinkhasov A. Long COVID, the Brain, Nerves, and Cognitive Function. Neurol Int. 2023 (9)
Proposed Mechanisms underlying Neurological Symptoms
Direct viral impact and neuro-invasion.
The virus can enter the brain via the olfactory bulb or the blood stream leading to neuroinflammation, neuronal damage and impaired brain function. The SARS-CoV-2 can persist in brain tissue with alterations to neurons and glial lining of cerebral blood vessels. Encephalitis and focal brain injuries may or may not be reversible or connect to vascular changes in the brain. (24)
Strokes, migraine, movement disorders from tremor to involuntary muscle contractions, epilepsy, hearing and vision abnormalities, balance and coordination impairment as well as symptoms of Parkinson’s Disease, Alzheimer’s Disease, Multiple Sclerosis can occur. These are often multifactorial with genetic and environmental factors acting in concert.(25)
Direct neuro-invasion along with cytokine storm and neuroinflammation leads to nerve injury.
Neuronal and glial degeneration can occur in patients with COVID-19 regardless of overt clinical neurological manifestations. (26)
Pathological processes in neural tissue occur through a concerted breakdown in neuron-glia homeostasis, spanning neuron axonal damage, astrogliosis, microgliosis, and impaired neuron-glia communication. (27)
Disruption of Blood-Brain Barrier (BBB). The virus and associated inflammatory response can disrupt the BBB, facilitating viral neuro-invasion.
Oxidative Stress. This reflects an imbalance between the systemic manifestation of reactive oxygen species and the body’s ability to detoxify the reactive intermediates or repair the resulting damage.(28)
Immune response and Inflammation. The excessive immune response, the “cytokine storm” with excessive cytokines IL6 and TNF-α with microglial activation, cross-talk with astrocytes and mast cells, with systemic inflammation including neuro-inflammation, disruption of the blood-brain barrier and cognitive deficits.(29)
Mast cell activation. Both Mast Cell Activation Syndrome (MCAS) and Long Covid are characterized by cognitive impairment. It is theorized that SARS-CoV-2 may exaggerate pre-existing MCAS or could activate normal mast cells via persisting viral particles.
Neuroinflammation -when mast cells are activated they release inflammatory mediators eg cytokines and chemokines that can cross the BBB and increase brain inflammation and cognitive impairment.
Irrespective of the predominance of TLR activation, there is a cross talk between mast cells, astrocytes and microglia.(Figure 1)
Dysregulation of the Renin-angiotensin system (RAS), specifically through its receptor ACE2 may contribute to cognitive symptoms. The ACE2 enzyme plays a role in the brain in neuroprotection and cognitive functions, as seen in Alzheimers Disease. (30)
Hypoxia and vascular damage can result in strokes and other brain injury. Viral-induced thrombo-inflammatory mechanisms include:
Increased TLR expression and TLR-mediated platelet activation during COVID -19 appears to enhance vascular and coronary thrombosis. (31)
Activation of Platelets and Endothelial Cells. Platelets play a dynamic role, responding to endothelial damage and immune interaction.(32) Platelet TLR2 activation promotes thrombosis, while endothelial cell activation leads to vascular dysregulation.(33) SARS-CoV-2 can directly activate platelets leading to proinflammatory cytokine release and thrombus formation which contributes to endothelial damage by activating immune cells and the complement system.
TLR4 activation on platelets can promote the release of pro-inflammatory mediators contributing to thrombo-inflammation.
Vasculitis. SARS-CoV-2 promotes inflammation in blood vessels which can disrupt blood flow and cause tissue damage.
Direct infection of endothelial cells (34)
Immune-Mediated Mechanisms. Formation of immune complexes, activation of complement pathways and production of autoantibodies lead to inflammation and damage to vessel walls. (34)
Systemic Inflammation. The hyperinflammatory state with elevated cytokines and other inflammatory markers can cause further damage.(34)
Other manifestations include:
Cutaneous vasculitis with skin involvement from small vessel vasculitis
Systemic vasculitis with effects on various organ systems including kidneys, lungs and GI Tract
Kawasaki Disease-like Syndrome
TLR Activation of Endothelial Cells.
TLR2 is expressed on Endothelial Cells, and promotes pro-clotting immune cell recruitment -cytokines and chemokines, and contributes to tumour growth and vascularity. TLR2 endothelial cell activation leads to vascular dysregulation.(33)
TLR4 on Endothelial Cells. TLR4 is a central pattern recognition receptor in endothelial cells- it is more associated with potentiation of platelet responses to other stimuli and induction of a broad pro-inflammatory response in endothelial cells. They initiate downstream signalling pathways leading to release of cytokines, type 1 interferons and other pro-inflammatory mediators that can result in modulation of vascular tone, promotion of neutrophil trafficking, activation of coagulation pathways and increased vascular permeability.(35)
Indirect effects associated with the autonomic changes.
Over 70% of Long-COVID have cardiovascular autonomic disorder, 30% of these with POTS (Postural Orthostatic Tachycardia Syndrome) (36) TLR4 is expressed on microglia, the resident immune cells of the central nervous system. Activation of TLR4 on microglia triggers a cascade of inflammatory responses, notably IL6 and TNFα, which can lead to neuronal damage and contribute to the development of neurodegenerative diseases.(37)
The pathophysiology of the small fibre neuropathy (SFN) that refers to the increased “sensitization” may involve direct viral damage, immune-related mechanism, or combinations of both. . This is closely associated with the TLR2/astrocyte/glutamate sensitization.
Inhibition of this signalling can shift the microglia from a pro- to an anti-inflammatory state, protecting neuronal cells against cytotoxicity.
Astrocyte damage and activation of glutamate pathway. The TLR2/astrocyte/glutamate pathway plays a vital role in cognitive impairment through a series of interconnected mechanisms involving neuroinflammation, astrocyte dysfunction and glutamate dysregulation.
Astrocytes are glial cells performing numerous functions, maintaining homeostasis, providing support to neurons and regulating neurotransmitter systems. In the neuroinflammation of TLR2 activation astrocyte function is impaired, characterized by a reduced capacity of astrocytes to uptake and recycle glutamate, a critical neurotransmitter in the CNS.(38)(39)(40)
Glutamate is the primary excitatory neurotransmitter in the CNS, and its levels are normally tightly regulated by astrocytes. It has critical roles in multiple brain functions including memory formation and synaptic plasticity (the ability of neurons to change the strength of their connections, an important neurophysiological process in brain networks after any damage.)
Glutamine synthetase is an enzyme in astrocytes that breaks down glutamate into glutamine.(26) In excess glutamate has been linked to many neurodegenerative diseases eg Alzheimer’s Disease. (41)
In COVID, astrocyte dysfunction leads to accumulation of glutamate in the extracellular space which can interfere with normal neurotransmission and lead to glutamate excitotoxicity where excessive glutamate accumulation leads to neuronal damage and death.(27)(28)
Astrocyte/glutamate dysregulation has been considered a cause of observed hypometabolism in COVID-related brain fog, as observed on brain Spect scans (and seen also in brainstems in CFS).(40) This is complicated by our clinic findings in POTS Spect scans that demonstrate similar patterns of hypoperfusion and hyperperfusion (which reflects endothelial inflammation and impaired BBB), but from mechanical causes and vasoconstriction from autonomic activation primarily from nor-adrenalin, revealing the complex systems that need to be resolved to tackle the cognitive impairment and fatigue.
Astrocytes, with their endfeet enveloping the cerebral blood vessels, play a pivotal role in glymphatic function, facilitating the exchange between cerebrospinal fluid (CSF) and interstitial fluid (ISF) alongside perivascular spaces.
Griffith University have shown that transient receptor potential (TRP) ion channels in astrocytes play a crucial role in regulating astrocyte calcium signalling which in turn can affect the contraction and relaxation of astrocyte endfeet. Dysregulated TRP channel activity, as might occur via aberrant TLR signalling, could impair the dynamic regulation of astrocytic endfeet leading to compromised glymphatic clearance leading to compromised glymphatic clearance.
Astrocytes undergo complex morphological, biochemical, and functional remodelling aimed at mobilizing the regenerative potential of the central nervous system. If the brain is not directly damaged, resolution of systemic pathology usually results in restoration of the physiological homeostatic status of neuroglial cells. (11)
Psychological effects -ASD, ADHD, anxiety, depression and PTSD.(42)
There can be direct viral damage, systemic inflammation, psychological stress and the long-term effects of severe illness
Dysregulation of astrocytes and glutamate can lead to psychological symptoms.
Steardo et al (11) hypothesized that the neuropsychiatric consequences of COVID-19 are from maladaptive glial recovery. In a subset of patients, glial cells fail to recover after infection thus promoting the onset and progression of COVID-19-related neuropsychiatric diseases. There is evidence from post-mortem examinations of the brains of COVID-19 patients of alterations in both astrocytes and microglia.
Glutamate dysfunction is a known accompaniment in Autism Spectrum Disorder (43), ADHD (44)(45), fibromyalgia, migraine and others. It is not surprising these problems are increasingly overt after a COVID infection.
Elevated glutamate has been found in the thalamus and cortex in migraineurs which is thought to play a role in the hyperexcitability and sensitization increasing pain perception in migraine.(41)(46)
Elevated glutamate has been found in fibromyalgia in the insula or insular cortex, areas involved in pain and emotion. The glutamate elevation is thought to cause the pain amplification from glutamate-induced excitotoxicity and altered pain processing.(47)
Glymphatic dysfunction. (Described in Glymphatic System) (48)
The Glymphatic system is the brain’s sewer, clearing toxins, and distributing non-waste products
Glymphatic flow is very important when fatigue and cognitive impairment are present
Glymphatic dysfunction and neuroinflammation are 2 key risk factors for neurodegeneration
Disruptions in the astrocyte water channel AQP4 function can impair glymphatic clearance and lead to the accumulation of neurotoxic proteins like amyloid-beta and alpha-synuclein (49)
Function is affected by impaired intracerebral arterial flow and sleep disorder
Function affected by Covid via TRP pathway. TLR2 activation of the TRP pathway are thought to impact on astrocyte function, including changes in the morphology and contractility of astrocytic endfeet, thus reducing the glymphatic system’s efficiency
Elevated levels of glutamate can lead to excitotoxicity, contributing to neuronal and astrocytic damage and further exacerbate astrocyte dysfunction, affecting glymphatic flow
Physical “drivers” can impact on function especially neck posture –phones and computer usage in particular
Lymphatic obstruction appears to be critical in increasing CSF pressure. This usually improves with good posture, management of Thoracic Outlet Syndrome and Jugular Outlet Syndrome if present
LDN (low dose naltrexone) can improve Natural Killer Cell and glymphatic function. (50)
The Glymphatic Connection
Figure 4. The Glymphatic System

Source: Mogensen et al. The Glymphatic System (en)during Inflammation (19)
“Cerebrospinal fluid flows into the paravascular space around cerebral arteries, combining with interstitial fluid and parenchymal solutes, exiting down venous paravascular spaces. Exchange of solutes is driven primarily by arterial pulsation and regulated during sleep by the expansion and contraction of brain extracellular space.(19)”
The glymphatic systems in the brain and eye export fluid and solutes from metabolically active neural tissue. Fluids from both the brain and the eye drain via the cervical lymph vessels, which empty into the venous system at the level of the subclavian veins.
Natale et al (20) describe that disruption of the glymphatic system plays a crucial role in age-related brain dysfunction, and there is strong evidence documenting the clearance of b-amyloid and tau via this system, as well as potentially harmful metabolites. In obstructive sleep apnoea they describe increasing cerebral aggregation and increased neurodegeneration. Sullen et al (51) describe traumatic brain injury, and its involvement in sleep disorders and in increased accumulation of beta amyloid (Aβ) and phosphorylated tau (ptau) in the paravascular spaces and along interstitial pathways in chronic traumatic encephalopathy, related to impaired glymphatic function.
In haemorrhagic stroke, fibrin and other blood products occlude perivascular spaces, while “in ischaemic stroke there is an impaired CSF inflow and the release of several pro-inflammatory cytokines.” They also describe how an altered glymphatic function may account for idiopathic normal pressure hydrocephalus. “These pathological conditions are associated with a decrease in CSF influx to the glymphatic pathway or reduced clearance efficacy.”(51)
Schain et al (52) demonstrated “ that cortical spreading depression (CSD), an animal model of migraine aura, closes the paravascular space (PVS) and impairs glymphatic flow, which also implicates the glymphatic system in the altered cortical and endothelial functioning of the migraine brain.” CSD, a known instigator of migraine, produced a dramatic alteration in both the structure and function of the glymphatic system.
Schain et al’s (52) key findings were that cortical spreading depression produces:
a rapid closure of the paravascular space (PVS) around both arteries and veins on the pial surface of the cerebral cortex lasting several minutes, and gradually recovering over 30 min. They found a mismatch between the constriction or dilation of the blood vessel lumen and the closure of the PVS suggesting that this closure is not likely to result from changes in vessel diameter.
this closure is accompanied by a reduction in the outflow of interstitial fluid from the parenchyma into the PVS, reducing glymphatic flow.(52)
Their finding of decreased glymphatic flow is potentially of significance for understanding the long-term effects of migraine aura on brain health. It appears to provide a new framework for understanding the variety of structural and functional alterations seen in the migraine brain.(52)
“Another potential risk to the migraine brain arises from our observation that CSD causes a transient spike in the concentration of interstitial solutes in the space between the smooth muscle and endothelial wall. Given that some of these solutes may include inflammatory molecules, the repeated occurrence of such events could contribute to endothelial dysfunction in pial and cortical arteries.”(52)
Patients with migraine aura exhibit elevated levels of biomarkers of coagulation activity, inflammation, and oxidative stress, as well as enhanced arterial stiffness and changes to the vascular tone. (52)
Natale et al (61) describe “the glymphatic pathway is connected to a classic lymphatic network, associated with dural meninges covering the brain, as well as sheaths of cranial nerves, or drains via the olfactory route, then exiting through cranial foramina. This drains ultimately to deep and superficial lymph nodes.” They explain that “during ageing meningeal lymphatic vessels exhibit decreased vessel diameter and reduced drainage to cervical lymph nodes. Experimental studies in mice showed that ablated or ligated meningeal lymphatics led to an increase in b-amyloid deposition and macrophage recruitment to plaque sites, with a reduced extracellular clearance of altered proteins.”(61)
They also describe how an altered glymphatic function may account for idiopathic normal pressure hydrocephalus. “These pathological conditions are associated with a decrease in CSF influx to the glymphatic pathway or reduced clearance efficacy.” (61) Mouse studies have confirmed clearance from the meningeal lymphatics into the cervical lymphatic chains. (64)
Natale et al (20) describe how “not only the level of consciousness, but also body posture contributes to drainage.” Lymphatics of the face and head drain inferiorly into the pericervical lymphatic collar. This collar consists of a series of connected lymph nodes, which form a chain that encircles the junction of the head and the neck. The collar consists of the following groups of nodes (from posterior to anterior): occipital, postauricular (retroauricular), preauricular, submandibular, and submental.
Perineural spaces surround the cranial nerves, including the vagus to provide some level of CSF drainage to peripheral lymphatics. Natale et al (20) describe how “some insights can be provided by the ocular glymphatic system.” “Retrograde CSF inflow to the paravascular spaces in the optic nerve and eye to CSF pathway supports clearance of waste products from the retina and vitreous. This occurs in the opposite direction compared to CSF drainage, and neural activity seems to play a role on the rate of fluid fluxes, as light stimulation promotes fluid drainage and b-amyloid clearance….via the paravenous space and subsequently drained to lymphatic vessels.(20)
Fatigue
Fatigue, while accompanying the mitochondrial dysfunction/ oxidative stress, it can also be part of the secondary effects of the cytokine storm. Areas that must be considered in management:
Table 1: Potential sources of Chronic Fatigue
Mitochondrial dysfunction /oxidative stress. There are mitochondrial and inflammatory deleterious bad gene variants that are critical to mitochondrial dysfunction and the exaggerated immune response caused by COVID, that can only be demonstrated in DNA analysis
Reactivation of EBV and similar viruses
Hypoperfusion with mitochondrial dysfunction /oxidative stress (CFS and coathanger pain with progressive muscle depolarization)(53)
Astrocyte /glymphatic dysfunction (TLR2-driven or crosstalk with microglia/mast cells)
Neurotransmitter dysfunction -Dopamine/serotonin/glutamate pathways
Intracranial pressure abnormality with HPA axis dysfunction
Direct cardiac damage (eg pericarditis, myocarditis, reduced Ejection Fraction)
Pulmonary damage- embolic, inflammatory, malignancy
Renal dysfunction through multiple causes including renal vein thrombosis and nephrotic syndrome
Liver disease, with reduced clearance of fibrin degradation products
Undiagnosed malignancy that can accompany Natural Killer Cell dysfunction.(55)(56)(57)
Metabolic pathway abnormality/ DNA mutations /Dietary triggers. These include PEMT mutations and dysfunction in phosphatidycholine and phosphatidylserine metabolism where there are no biomarkers.(54)
Other vital DNA mutations associated with dysfunction include APO E4 with its known increased cardiovascular, neurodegenerative and liver dysfunction potential.
Small fibre neuropathy
Autonomic instability eg POTS, dysautonomia, orthostatic hypotension
Impaired cardiac function
Very common (again IL-6 and TNFa)-accompanying exaggerated neuropathy, rheumatoid arthritis, reactive arthritis, PMR strongly suggestive of TLR4 mutations
Cardiac preload failure (unexplained shortness of breath with postural change)
Locus coeruleus
Aberrant azygous anatomy/ sympathetic activation (currently being investigated)
Sensitization mechanically
Secondary to Thoracic /Lymphatic duct obstruction
Associated with Intra-abdominal vascular dysfunction
DNA Mutations:
From the DNA studies by Dr Valerio Vittone,(18) the mutations in the mast cell membrane and in Dao enzyme function are major contributors to the pathogenesis of Long COVID, and when combined with his other findings, clear paths are emerging to reduce the severity of COVID infections and in the management of Long COVID. Problems such as persisting thromboinflammation as may be seen in persistently elevated D-Dimer may be identified with DNA as there are no available biomarkers for many of these mutations.
Using a technique called genetic imputation, it is now possible to use the ~750,000 SNPs on a chip into up to 83 million additional SNP variants with an accuracy rate of 98%.
More than 400 genes are differentially expressed in long covid patients. Primary problems from our DNA appear to be in the following, and dealt with in more detail in “DNA Mutations that Underpin POTS and Long COVID.”)(18): Ongoing DNA assays in Long COVID that confirm similar findings warrant further investigation in a broader cohort of Long COVID subtypes.
There is increasing DNA evidence that multiple mutations in the Toll-Like Receptors (especially “first responders” TLR2 and TLR4) play a large role in the individual immune response, and associated with “downstream” mutations can create a domino effect responsible for the individual problems being caused by Covid. These are common and look to provide directions for management in patients not responding to the first line mast cell blockade.
The TLR4 mutations appear to be potentially one of the critical “molecular connections” associated with the abnormally low “biomarkers” used to measure the inflammatory responses seen in many Covid patients. These patients have often “fallen below the radar” while in fact have an impaired immune response.
When looking at recovering Long Covid patients, there are mutations in mast cell function Dr Vittone has discovered, with one on the mast cell membrane. Furthermore, Dr Vittone, correlated additional mutations of two critical enzymes (namely DAO & HNMT) involved in clearing histamines in different tissues in the body.
The Dao enzyme (Diamine oxidase) is involved in the metabolism, oxidation, and inactivation of histamine and other polyamines such as putrescine or spermidine. DAO is produced in kidneys, thymus and intestinal lining that breaks down excess histamine in the body, particularly in the gut.
HNMT Histamine N-methyltransferase) catalyzes the methylation of histamine in the presence of S-adenosylmethionine (SAM-e) forming N-methylhistamine. HNMT is involved in metabolism of intracellular histamine, mainly in kidneys and liver, but also in bronchi, large intestine, ovary, prostate, spinal cord, spleen, trachea. and peripheral tissue.
Vascular complications in COVID-patients have been linked phosphatidylserine to thrombo-inflammation. Thrombo-inflammation plays a critical role through complement activation and cytokine release, platelet overactivity, apoptosis (thrombocytopathy), as well as coagulation abnormalities (coagulopathy).(17)(58) Phosphatidylserine is associated with increased thrombo-inflammation and vascular complications, and can trigger the blood coagulation cascade, the complement system, inflammation and reside on activated immune cells. Phosphatidylserine is synthesized from phosphatidylcholine through a Ca2+ -dependent reaction in the endoplasmic reticulum. PEMT mutations, as well as other thrombophilia-related genes eg Factor V Leidon are likely to be associated with persisting elevated D-Dimer levels in Long COVID
COVID-19 severity is associated with immune abnormalities such as increased inflammatory cytokines, immune cell exhaustion, and general lymphopenia. Hyperactivated platelets, elevated levels of circulating PMPs, and an increased risk of thromboembolic complications - related to phosphatidylserine -associated immune mechanisms - contribute to COVID-19 disease severity and death.(17)
Toll-Like Receptors (especially “first responders” TLR2 and TLR4) play a large role in the individual immune response, and associated with “downstream” mutations can create a domino effect responsible for the individual problems being caused by Covid.
TLR2 senses the SARS-CoV-2 envelope protein (E), resulting in production of inflammatory cytokines and chemokines, contributing to the hyperinflammatory state and tissue damage seen in severe Covid. The severity of the Covid infection is largely determined by the E Protein /TLR2 activation rather than the S protein.(6)
TLR4 signalling is activated by the Spike protein (S). This can lead to a pro-thrombotic and pro-inflammatory state contributing to severe complications eg myocardial infarction and acute lung injury.(8)(9).
The endosomal TLR3 senses intracellular viral dsRNA. Activated TLR regulates the production of proinflammatory factors through a series of signalling in the NF‐κB pathway and activates IRF3/7 to produce I IFN. (6)(90) A DNA variant in TLR3 has also been identified as increasing susceptibility and mortality to acute COVID infections by decreasing TLR3 expression and impairing recognition of SARS-Co-V dsRNA. (6) These results suggest perivascular inflammation may be a critical factor in Long COVID, but the role of these receptors in Long COVID associated POTS has not been established.
Mast cell mutations that affect body’s ability to respond to mast cell activation and threats mediated through mast cells. These are major mutations in POTS and thus far in Long Covid. The primary ones are on the mast cell membranes and in the function of Dao and HNMT enzyme. Mast cells play a key role in homeostatic mechanisms and surveillance, recognizing and responding to different pathogens, and tissue injury. An abundance of mast cells reside in connective tissue that borders with the external world (the skin as well as gastrointestinal, respiratory, and urogenital tracts.)
COMT mutations (= reduced ability to process catecholamines and oestrogen with implications in malignancy, rheumatoid arthritis and SLE) Catechol-O-Methyltransferase (COMT) is one of several enzymes that degrade catecholamines eg dopamine, adrenaline, nor-adrenaline, catecholestrogens and various drugs. COMT introduces a methyl group to the catecholamines which is donated by S-adenosylmethionine (SAM). (59) Therefore you need adequate SAM for COMT to work. Having too little SAM and too much SAH (s-adenosylhomocysteine) from undermethylation results in COMT inhibition as well. (60) For this reason, MTHFR SNPs that cause undermethylation and COMT SNPs that lower COMT levels are a bad combination. (61)
COMT gene production is itself influenced by methylation. (62) Usually, methylation shuts down gene production.
Oestrogen also signals mast cells to release histamines via its ER receptor on Mast cells. This is a critical mutation in POTS. COMT gene production is itself influenced by methylation (in the presence of SAMe a product of the methylation cycle), which in turn is affected by multiple mutations, some very common such as the MTHFR genes.
There is an association between COMT mutations with malignancy especially breast cancer, and also endometriosis and auto-immune disease. Then there are various oxidative stress and inflammatory mutations. These combinations are amenable to epigenetic modification Dr Vittone can provide with a personalized protocol based on individual genetics.
Oxidative stress and mitochondrial mutations- eg eNOS, SOD2. NO metabolism- associated with the development of FMS and pain sensitization, The pain sensitization is also influenced by the astrocyte/glutamate dysfunction.
Inflammatory mutations- various mutations in interleukins.
Methylation mutations (especially MTHFR) the 677 MTHFR mutation typically is associated with increased homocysteine, and affects collagen function via SAMe and other molecules as well as increased thrombotic risk and plaque formation in different tissues.
Phosphatidylethanolamine N-methyltransferase (PEMT) catalyzes phosphatidylcholine synthesis. It is thought that PEMT gene polymorphisms are associated with non-alcoholic fatty liver disease (NAFLD).(63) Altered phospholipid metabolism is thought to sensitize mast cells to activation or affect their response to threats.(64) Fatigue is a common symptom of PEMT mutations and its associated mitochondrial dysfunction. The role of PEMT in COVID thrombo-inflammation is discussed above.
The CHKA gene codes for the choline kinase alpha enzyme, which is involved in the pathway of reactions that coverts choline into phosphatidylcholine needed for cell membranes.
PEMT and similar mutations may provide the missing link to the non-resolving elevated D-Dimer measurements for microemboli, as well as persisting cognitive impairment despite mast cell blockade. There are no tests currently available to test for this except DNA, and even then a decision needs to be made about long term supplementation, as this mutation is associated with neurodegeneration, as with APO E4. The addition of SPECT scanning of the brain is being used to assess hypo-and hyperperfusion in Long Covid, and is of value in managing these patients.
BHMT, PEMT and CHKA are connected to MTHFR and the folate and methylation cycle so mutations in genes like MTR and MTFHR indirectly affect them too, eg methyl folate is used by BHMT to convert betaine into methionine.
TRP mutations- TRPM3 appears critical in NK (Natural Killer) immune cell function, with implications for Ca2+ signalling and cell function.(65) The transient receptor potential melastatin subfamily 3 (TRPM3) is one of the most primitive receptors in the body, activated by a wide variety of agents, from bacteria and viruses to temperature and environmental factors such as perfumes. This diversity made it a logical suspect for a condition like CFS that has so many different triggers in different people.
Prof Sonya Marshall-Gradisnik and the Griffith University Chronic Fatigue team working with TRPM3 function in the research into chronic fatigue at Griffith University (66)(50)have linked mutations in this pathway with “glymphatic” function with consequent reduced clearance of waste solutes from the brain with production of fatigue and brain fog and the therapeutic benefit of Low Dose Naltrexone
TRPA1 is a key ion channel that detects oxidative stress and a range of endogenous and exogenous chemicals (smoke, solvents, cold air).
TRPM3 activity is impaired in CFS/ME patients suggesting changes in intracellular Ca2+ concentration, which may impact NK cellular functions. This investigation further helps to understand the intracellular-mediated roles in NK cells and confirm the potential role of TRPM3 ion channels in the aetiology and pathomechanism of CFS/ME.(66)(67)(50)
NLRP3 mutations look to play a major role in this neurodegenerative/inflammatory process as University of Qld researchers Woodruff et al demonstrated that SARS drives NLRP3 inflammasome activation in human microglia through spike protein.
Lipoprotein a (LPa): High levels of LPa, found in 20% of European descent, increases the inflammatory response and thrombotic risk in COVID-19. Thrombotic activity marked by elevated D-Dimer was associated with higher LPa.(68)
APO E4 - The Apolipoprotein E allele 4 is a major genetic risk factor for Alzheimer's disease, as this lipid carrier is important for maintaining homeostasis necessary for a healthy environment of the brain. This mutation is seen in around 15 to 20% of the general population, with 2-3% being homozygous with the increased risks that are associated. This is emerging as a significant mutation in resistant cognitive impairment. Of note there has been research into the use of ARB medication candesartan and telmisartan in reducing risk- this is the subject under close scrutiny, particularly with increasing evidence of neuroprotection afforded by candesartan and telmisartan.
APO E is particularly concentrated in astrocytic processes at the pial surface and around the blood vessels. In addition, the choroid plexus and tanycytes in the wall of the third ventricle also produce Apolipoprotein E. Thus, Apolipoprotein E production is co-localized with CSF production sites and transport pathways suggesting that lipids are transported by the glymphatic system.
The glymphatic system is thought to play a central role in macroscopic distribution of lipids in the brain and that medium to large lipid soluble molecules might require carrier particles in order to be delivered via the CSF. Astrocytes thus play a key role in lipid synthesis and lipid distribution by releasing lipid carrier proteins, such as Apolipoprotein E, and in maintaining the highway for distribution, the glymphatic system.
APO E4 mutation also affects arteries, significantly increasing coronary artery disease risk ,decreased mitochondrial function, decreased insulin sensitivity, increased insulin resistance, fatty liver and progression to cirrhosis (APO E4 contributing to altered VLDL metabolism and increased atherosclerosis).(69)
A single mutation, by itself, is insufficient to cause the symptoms of FMS, POTS or Long Covid. It is recognition of DNA patterns so as the sensitization from microglial activation is reduced, we can work with management protocols based on DNA mutations to counter residual problems and look for patterns that may impact on future health.
Vaccine Activation:
Brisbane Immunologist Prof Robert Tindle (70) describes in the Australian Journal of General Practice that there is concern that COVID-19 vaccination per se might contribute to long COVID. COVID-19 vaccines utilise a modified, stabilised prefusion spike protein that might share similar toxic effects with its viral counterpart.(71)(72) He describes how a possible association between COVID-19 vaccination and the incidence of POTS has been demonstrated in a large study, though at a rate that was one-fifth of the incidence of POTS after SARS-CoV-2 infection. (73)
He described multiple studies having shown an increased risk of myocarditis after vaccination with mRNA encoding SARS-CoV-2 spike protein. (74)(75)(76) Spike protein expression has been found in muscle tissue, the lymphatic system, cardiomyocytes and other cells after entry into the circulation.(75)
Recipients of two or more injections of the mRNA vaccines display a change in immune response to IgG4 antibodies, where there may be an association with autoimmune diseases, increased cancer growth, autoimmune myocarditis and other IgG 4-related diseases (IgG4-RD) in susceptible individuals. (77)
He describes the need for understanding the persistence of viral mRNA and viral protein and their cellular pathological effects after vaccination with and without infection . Because COVID-19 vaccines were approved without long-term safety data given the urgent need for a vaccination to halt the rapidly escalating pandemic, it is perhaps premature to assume that past SARS-CoV-2 infection is the sole common factor in long COVID.(70)
Co-morbidity Overlap
Long Covid research confirms symptom overlap between CFS and Long Covid both in symptom burden and exercise derangements, through impaired cardiac preload and peripheral oxygen extraction, associated with autonomic dysfunction, small fibre neuropathy, ganglionopathy, and mitochondrial dysfunction.(78)
“Coat hanger pain” is seen frequently in patients with dysautonomia, especially POTS, Long Covid, as well as fibromyalgia and CFS. Humm et al (53) describes the typical ache usually began with standing or sitting, as discomfort and pain in the neck and shoulder, and research suggests this could result from reduced blood flow and oxygen delivery to the muscles in the affected areas. They showed that muscle membranes in patients with orthostatic hypotension become progressively depolarized during standing, which they felt was most likely the result of muscle ischaemia, related to the drop in perfusion pressure caused by orthostatic hypotension.
The theory on this was proposed over 16 years ago when pain could be induced by vasomotor dysregulation, and vasoconstriction in muscle, leading to low-level ischaemia and its metabolic sequelae. Vasodilatory influences, including physical activity, relieve the pain of FMS by increasing muscle perfusion,(79) confirming the importance of paced appropriate physical activity.
Hypoperfusion in the brainstem is a very common finding in brain SPECT scans in CFS. Wirth et al (80) described reduced blood flow causing neurological symptoms including impaired cognitive function or “brain fog.” Increased intracranial pressure, as proposed by Hulens (82) and Bragee (81) has also been frequently observed compounding problems.
The brainstem hypoperfusion seen in CFS Spect scans is believed to be part of the same process of hypoperfusion and mitochondrial dysfunction that underpins the coat hanger pain of FMS,(53) although an emergent hypothesis of glutamate/astrocyte dysfunction by Guedl (40) in Long Covid provides a possible explanation for astrocyte/glutamate -induced impaired glymphatic function through impairment of the paravascular space function and potential effects on symptoms such as fatigue, brain fog and head pressure.
Wirth et al (80) noted that reduced cerebral blood flow in CFS can lead to a range of neurological symptoms, including psychomotor slowing and impaired cognitive function in CFS. This hypoperfusion can impact both the global and local regulation of blood flow in the brain. They also reported an increase in intracranial pressure has been observed in ME/CFS patients. In “heads-up” tilt testing, CFS patients had a 26% reduction in cerebral blood flow (against 7% in normal.) In the severe CFS, a reduction in blood flow from lying to sitting was 24.5%. As a possible explanation for the orthostatic intolerance and the decrease in cerebral blood flow they proposed the presence of both a strong vasoconstrictor effect mediated by an elevated sympathetic tone and weakened vasodilator influences. Covid-19 seriously affects the endothelium and there is evidence of chronic endothelial dysfunction in the post-Covid-syndrome similar to that in ME/CFS.(80)
Wirth et al (80) also reported muscle mitochondrial dysfunction, as evidenced by higher levels of pyruvate and lower levels of ATP and phosphocreatine in muscles, suggesting an impairment in muscle energy metabolism, which is also observed in ME/CFS, indicating a likely overlap in the pathophysiological mechanisms of these conditions.
Long COVID research has also shown post-exertional malaise (PEM), with associated fatigue, pain and local and systemic metabolic disturbances, severe exercise-induced myopathy and tissue infiltration of amyloid-containing deposits in skeletal muscles of patients with long COVID.(83) While the amyloid may not be present in the FMS, the underlying DNA and mechanical “drivers” are all much the same.
Research from Germain et at (84) demonstrated a shift in metabolism towards amino acids as an energy source as an association with Post-Exertional Malaise, with glutamate in particular involved. The changing levels of amino acids are currently being explored in our clinic setting.
A number of abnormalities in pain processing have been demonstrated in fibromyalgia. Among them are the following:
Excess excitatory (pronociceptive) neurotransmitters (eg, substance P, glutamate levels in the insula)
Low levels of inhibitory neurotransmitters (eg, serotonin and noradrenalin) in descending antinociceptive pathways in the spinal cord
Maintained enhancement of temporal summation of second pain
Altered endogenous opioid analgesic activity in several brain regions known to play a role in pain modulation
Dopamine dysregulation (85)
The biochemical changes seen in the CNS, the low levels of serotonin, the four-fold increase in nerve growth factor, and the elevated levels of substance P all lead to a whole-body hypersensitivity to pain and suggest that fibromyalgia is a condition of central sensitization and abnormal central processing of nociceptive pain input.
There is evidence that interactions within the brain and gut axis (BGA) that involves both, the afferent- ascending and the efferent-descending pathways as well as the somatosensory cortex, insula, amygdala, anterior cingulate cortex and hippocampus are deranged in IBS showing both the activation and inactivation.(86) Alterations in the bi-directional signaling between the enteric nervous system and the central nervous system and consequently between the brain and the gut may play a significant role in the pathophysiology of IBS.(86)
Low grade inflammation has been implicated as one of the underlying mechanisms of IBS. Variations in the circulating pro-inflammatory interleukin-6 (IL-6) levels and IL-6 gene polymorphisms have been demonstrated in IBS. Basasharti et al (87) found levels of pro-inflammatory interleukins 2,6 and 8 have been found to be elevated in IBS, especially in the post-infectious IBS (against non-post-infectious IBS) and reduction of anti-inflammatory IL-10 in both.
The most widely acknowledged biochemical abnormality associated with fibromyalgia is abnormally low serotonin levels. Many studies have linked serotonin, a neurotransmitter, to sleep, pain perception, headaches, and mood disorders.(86) Dopamine has also been studied. While brain dopamine is best known for its role in pleasure, motivation and motor control, it is also involved in pain modulation, with an impaired response to pain stimuli.(88)
Serotonin and noradrenalin have been shown to have a mainly excitatory influence on acute stress responses and both are key in circadian rhythm of the HPA axis. Dopamine has excitatory influences on the basal tone of the HPA axis and enhances acute stress responses. Glutamate is present also in inhibitory stress circuits, and elevated levels are usually found in fibromyalgia, migraine, ADHD and GWS.(89) Levels in Long-COVID have not been established.
The presence of the pain that typifies the abnormal pain processing in fibromyalgia provides a pathway to the TLR2/astrocyte/glutamate dysfunction. As the research into POTS, FMS and Long COVID “drivers” following the activating of the syndrome through Mast Cell Activation continues, it is identification of the ongoing “drivers” that provides the best likely long term solutions. A genetic predisposition is unquestionable seen both in family clusters and the simple fact that most people who have the recognized “drivers” that have been found simply have no symptoms. These “drivers” may be from the gut, or mechanical commonly affecting the sympathetic chain or vagus, and their identification allows a targeted program which includes management of MCAS to be prepared for each POTS or Long-Covid patient.
References:
1. Cheetham NJ, Penfold R, Giunchiglia V, Bowyer V, Sudre CH, Canas LS, Deng J, Murray B, Kerfoot E, Antonelli M, Rjoob K, Molteni E, Österdahl MF, Harvey NR, Trender WR, Malim MH, Doores KJ, Hellyer PJ, Modat M, Hammers A, Ourselin S, Duncan EL, Hampshire A, Steves CJ. The effects of COVID-19 on cognitive performance in a community-based cohort: a COVID symptom study biobank prospective cohort study. EClinicalMedicine. 2023 Jul 21;62:102086. doi: 10.1016/j.eclinm.2023.102086. PMID: 37654669; PMCID: PMC10466229.
2. Afrin, Lawrence; Weinstock, Leonard; Molderings, Gerhard. Covid-19 Hyperinflammation and post-Covid 19 may be rooted in Mast Cell Activation Syndrome. 2020: International Journal of Infectious Diseases 100, 327-332.
3. Sarkar S, Karmakar S, Basu M, Ghosh P, Ghosh MK. Neurological damages in COVID-19 patients: Mechanisms and preventive interventions. MedComm (2020). 2023 Apr 6;4(2):e247. doi: 10.1002/mco2.247. PMID: 37035134; PMCID: PMC10080216.
4. Sashindranath, M., Nandurkar H. Endothelial Dysfunction in the Brain. AHA Journals. Stroke 2021. https://www.ahajournals.org/doi/10.1161/STROKEAHA.120.032711
5. Phulwani NK, Esen N, Syed MM, Kielian T. TLR2 expression in astrocytes is induced by TNF-alpha- and NF-kappa B-dependent pathways. J Immunol. 2008 Sep 15;181(6):3841-9. doi: 10.4049/jimmunol.181.6.3841. PMID: 18768838; PMCID: PMC2649826.
6. Mantovani S, Oliviero B, Varchetta S, Renieri A, Mondelli MU. TLRs: Innate Immune Sentries against SARS-CoV-2 Infection. Int J Mol Sci. 2023 Apr 29;24(9):8065. doi: 10.3390/ijms24098065. PMID: 37175768; PMCID: PMC10178469.
7. Sariol, A., Perlman, S. SARS-CoV-2 takes its Toll. Nat Immunol 22, 801–802 (2021). https://doi.org/10.1038/s41590-021-00962-w
8. Aboudounya MM, Heads RJ. COVID-19 and Toll-Like Receptor 4 (TLR4): SARS-CoV-2 May Bind and Activate TLR4 to Increase ACE2 Expression, Facilitating Entry and Causing Hyperinflammation. Mediators Inflamm. 2021 Jan 14;2021:8874339. doi: 10.1155/2021/8874339. PMID: 33505220; PMCID: PMC7811571.
9. van der Donk LEH, Bermejo-Jambrina M, van Hamme JL, Volkers MMW, van Nuenen AC, Kootstra NA, Geijtenbeek TBH. SARS-CoV-2 suppresses TLR4-induced immunity by dendritic cells via C-type lectin receptor DC-SIGN. PLoS Pathog. 2023 Oct 16;19(10):e1011735. doi: 10.1371/journal.ppat.1011735. PMID: 37844099; PMCID: PMC10602378.
10. Jiang, Yujie & Zhao, Tingmei & Zhou, Xueyan & Xiang, Yu & Gutierrez Castrellon, Pedro & Ma, Xuelei. (2022). Inflammatory pathways in COVID‐19: Mechanism and therapeutic interventions. MedComm. 3. 10.1002/mco2.154.
11. Steardo L Jr, Steardo L, Scuderi C. Astrocytes and the Psychiatric Sequelae of COVID-19: What We Learned from the Pandemic. Neurochem Res. 2023 Apr;48(4):1015-1025. doi: 10.1007/s11064-022-03709-7. Epub 2022 Aug 3. PMID: 35922744; PMCID: PMC9362636.
12. Carthy, Elliott & Ellender, Tommas. (2021). Histamine, Neuroinflammation and Neurodevelopment: A Review. Frontiers in Neuroscience. 15. 10.3389/fnins.2021.680214
13. Theoharides TC, Kempuraj D. Role of SARS-CoV-2 Spike-Protein-Induced Activation of Microglia and Mast Cells in the Pathogenesis of Neuro-COVID. Cells. 2023 Feb 22;12(5):688. doi: 10.3390/cells12050688. PMID: 36899824; PMCID: PMC10001285.
14. Bayat AH, Azimi H, Hassani Moghaddam M, Ebrahimi V, Fathi M, Vakili K, Mahmoudiasl GR, Forouzesh M, Boroujeni ME, Nariman Z, Abbaszadeh HA, Aryan A, Aliaghaei A, Abdollahifar MA. COVID-19 causes neuronal degeneration and reduces neurogenesis in human hippocampus. Apoptosis. 2022 Dec;27(11-12):852-868. doi: 10.1007/s10495-022-01754-9. Epub 2022 Jul 25. PMID: 35876935; PMCID: PMC9310365.
15. Ransohoff RM, Brown MA. Innate immunity in the central nervous system. J Clin Invest. 2012 Apr;122(4):1164-71. doi: 10.1172/JCI58644. Epub 2012 Apr 2. PMID: 22466658; PMCID: PMC3314450.
16. Mukherjee, R. et al. Famotidine inhibits toll-like receptor 3-mediated inflammatory signaling in SARS-CoV-2 infection. 2021. JBC Research Article. https://www.sciencedirect.com/science/article/pii/S0021925821007250
17. Rauch, L et al. Binding of phosphatidylserine-positive microparticles by PBMCs classifies disease severity in COVID-19 patients. 2021 bioRxiv. doi: https://doi.org/10.1101/2021.06.18.448935
18. Vittone,V, Exelby, G. DNA Mutations that Underpin POTS and Long Covid. 2023. https://www.mcmc-research.com/post/dna-mutations-that-underpin-pots-and-long-covid
19. Mogensen, F.L.-H.; Delle, C.; Nedergaard, M. The Glymphatic System (En)during Inflammation. Int. J. Mol. Sci. 2021, 22, 7491. https://doi.org/10.3390/ijms22147491
20. Natale,G et al. Glymphatic System as a Gateway to Connect Neurodegeneration from Periphery to CNS. 2021. Glymphatic System as a Gateway to Connect Neurodegeneration From Periphery to CNS. Front. Neurosci. 15:639140. doi: 10.3389/fnins.2021.639140
21. Pretorius,E. et al.: Persistent clotting protein pathology in Long COVID/Post‑Acute Sequelae of COVID‑19 (PASC) is accompanied by increased levels of antiplasmin. Cardiovasc Diabetol (2021) 20:172. https://doi.org/10.1186/s12933-021-01359-7
22. Pretorius et al.: Prevalence of Amyloid Blood Clots in COVID-19 in plasma. https://www.researchgate.net/publication/343313974_Prevalence_of_amyloid_blood_clots_in_COVID-19_plasma
23. Bell, D., Laubscher, G., Pretorius, E.: A central role for amyloid fibrin microclots in long COVID/PASC: origins and therapeutic implications. Biochem J (2022) 479 (4): 537–559. https://doi.org/10.1042/BCJ20220016
24. Granholm AC. Long-Term Effects of SARS-CoV-2 in the Brain: Clinical Consequences and Molecular Mechanisms. J Clin Med. 2023 Apr 28;12(9):3190. doi: 10.3390/jcm12093190. PMID: 37176630; PMCID: PMC10179128.
25. Xu E, Xie Y, Al-Aly Z. Long-term neurologic outcomes of COVID-19. Nat Med. 2022 Nov;28(11):2406-2415. doi: 10.1038/s41591-022-02001-z. Epub 2022 Sep 22. PMID: 36138154; PMCID: PMC9671811.
26. Plantone D, Locci S, Bergantini L, et al. Brain neuronal and glial damage during acute COVID-19 infection in absence of clinical neurological manifestations. Journal of Neurology, Neurosurgery & Psychiatry 2022;93:1343-1348.
27. Savelieff MG, Feldman EL, Stino AM. Neurological sequela and disruption of neuron-glia homeostasis in SARS-CoV-2 infection. Neurobiol Dis. 2022 Jun 15;168:105715. doi: 10.1016/j.nbd.2022.105715. Epub 2022 Mar 29. PMID: 35364273; PMCID: PMC8963977.
28. Betteridge DJ. What is oxidative stress? Metabolism. 2000 Feb;49(2 Suppl 1):3-8. doi: 10.1016/s0026-0495(00)80077-3. PMID: 10693912.
29. Reiss AB, Greene C, Dayaramani C, Rauchman SH, Stecker MM, De Leon J, Pinkhasov A. Long COVID, the Brain, Nerves, and Cognitive Function. Neurol Int. 2023 Jul 6;15(3):821-841. doi: 10.3390/neurolint15030052. PMID: 37489358; PMCID: PMC10366776.
30. Reveret, L., Leclerc, M., Emond, V. et al. Higher angiotensin-converting enzyme 2 (ACE2) levels in the brain of individuals with Alzheimer’s disease. acta neuropathol commun 11, 159 (2023). https://doi.org/10.1186/s40478-023-01647-1
31. Fard,M et al: Thrombosis in COVID-19 Infection: Role of platelet activation-mediated immunity. Thrombosis Journal (2021) 19:59 https://doi.org/10.1186/s12959-021-00311-9
32. Guo L, Rondina MT. The Era of Thromboinflammation: Platelets Are Dynamic Sensors and Effector Cells During Infectious Diseases. Front Immunol. 2019 Sep 13;10:2204. doi: 10.3389/fimmu.2019.02204. PMID: 31572400; PMCID: PMC6753373.
33. Guillevin L, Dörner T. Vasculitis: mechanisms involved and clinical manifestations. Arthritis Res Ther. 2007;9 Suppl 2(Suppl 2):S9. doi: 10.1186/ar2193. PMID: 17767747; PMCID: PMC2072881.
34. Giryes S, Bragazzi NL, Bridgewood C, De Marco G, McGonagle D. COVID-19 Vasculitis and vasculopathy-Distinct immunopathology emerging from the close juxtaposition of Type II Pneumocytes and Pulmonary Endothelial Cells. Semin Immunopathol. 2022 May;44(3):375-390. doi: 10.1007/s00281-022-00928-6. Epub 2022 Apr 12. PMID: 35412072; PMCID: PMC9003176.
35. Stierschneider A, Wiesner C. Shedding light on the molecular and regulatory mechanisms of TLR4 signaling in endothelial cells under physiological and inflamed conditions. Front Immunol. 2023 Nov 24;14:1264889. doi: 10.3389/fimmu.2023.1264889. PMID: 38077393; PMCID: PMC10704247.
36. Hira, R et al. Objective Hemodynamic Cardiovascular Autonomic Abnormalities in Post-Acute Sequelae of COVID-19. 2022. Canadian Journal of Cardiology. DOI: https://doi.org/10.1016/j.cjca.2022.12.002
37. Jin, S., Kim, J., Park, J. et al. Hypothalamic TLR2 triggers sickness behavior via a microglia-neuronal axis. Sci Rep 6, 29424 (2016). https://doi.org/10.1038/srep29424
38. Li L, Acioglu C, Heary RF, Elkabes S. Role of astroglial toll-like receptors (TLRs) in central nervous system infections, injury and neurodegenerative diseases. Brain Behav Immun. 2021 Jan;91:740-755. doi: 10.1016/j.bbi.2020.10.007. Epub 2020 Oct 8. PMID: 33039660; PMCID: PMC7543714.
39. Verkhratsky, A., Butt, A., Li, B. et al. Astrocytes in human central nervous system diseases: a frontier for new therapies. Sig Transduct Target Ther 8, 396 (2023). https://doi.org/10.1038/s41392-023-01628-9
40. Hotowitz,T, Pellurin,L, Zimmer, E, Guedj,E. Brain fog in long COVID: A glutamatergic hypothesis with astrocyte dysfunction accounting for brain PET glucose hypometabolism. Elsevier, Medical Hypotheses. https://doi.org/10.1016/j.mehy.2023.111186
41. Ronald Zielman, Jannie P. Wijnen, Andrew Webb, Gerrit L. J. Onderwater, Itamar Ronen, Michel D. Ferrari, Hermien E. Kan, Gisela M. Terwindt, Mark C. Kruit, Cortical glutamate in migraine, Brain, Volume 140, Issue 7, July 2017, Pages 1859–1871, https://doi.org/10.1093/brain/awx130
42. Hampshire A, Trender W, Chamberlain SR, Jolly AE, Grant JE, Patrick F, Mazibuko N, Williams SC, Barnby JM, Hellyer P, Mehta MA. Cognitive deficits in people who have recovered from COVID-19. EClinicalMedicine. 2021 Sep;39:101044. doi: 10.1016/j.eclinm.2021.101044. Epub 2021 Jul 23. PMID: 34316551; PMCID: PMC8298139.
43. Zheng Z, Zhu T, Qu Y, Mu D. Blood Glutamate Levels in Autism Spectrum Disorder: A Systematic Review and Meta-Analysis. PLoS One. 2016 Jul 8;11(7):e0158688. doi: 10.1371/journal.pone.0158688. PMID: 27390857; PMCID: PMC4938426.
44. Maltezos S, Horder J, Coghlan S, Skirrow C, O'Gorman R, Lavender TJ, Mendez MA, Mehta M, Daly E, Xenitidis K, Paliokosta E, Spain D, Pitts M, Asherson P, Lythgoe DJ, Barker GJ, Murphy DG. Glutamate/glutamine and neuronal integrity in adults with ADHD: a proton MRS study. Transl Psychiatry. 2014 Mar 18;4(3):e373. doi: 10.1038/tp.2014.11. PMID: 24643164; PMCID: PMC3966039.
45. Cheng, J., Liu, A., Shi, M. et al. Disrupted Glutamatergic Transmission in Prefrontal Cortex Contributes to Behavioral Abnormality in an Animal Model of ADHD. Neuropsychopharmacol 42, 2096–2104 (2017). https://doi.org/10.1038/npp.2017.30
46. Bathel, A., Schweizer, L., Stude, P. et al. Increased thalamic glutamate/glutamine levels in migraineurs. J Headache Pain 19, 55 (2018). https://doi.org/10.1186/s10194-018-0885-8
47. Harris RE, Sundgren PC, Craig AD, Kirshenbaum E, Sen A, Napadow V, Clauw DJ. Elevated insular glutamate in fibromyalgia is associated with experimental pain. Arthritis Rheum. 2009 Oct;60(10):3146-52. doi: 10.1002/art.24849. PMID: 19790053; PMCID: PMC2827610.
48. Exelby, G. The Glymphatic System. 2023. https://www.mcmc-research.com/post/the-glymphatic-system
49. Szlufik S, Kopeć K, Szleszkowski S, Koziorowski D. Glymphatic System Pathology and Neuroinflammation as Two Risk Factors of Neurodegeneration. Cells. 2024 Feb 5;13(3):286. doi: 10.3390/cells13030286. PMID: 38334678; PMCID: PMC10855155.
50. Cabanas,H, Muraki, K,Eaton-Fitch,N, Staines,D, Marshall-Gradisnik,S. Potential Therapeutic Benefit of Low Dose Naltrexone in Myalgic Encephalomyelitis/Chronic Fatigue Syndrome: Role of Transient Receptor Potential Melastatin 3 Ion Channels in Pathophysiology and Treatment. Frontiers in Immunology. 2021. https://www.frontiersin.org/articles/10.3389/fimmu.2021.687806/full
51. Sullan MJ, Asken BM, Jaffee MS, DeKosky ST, Bauer RM. Glymphatic system disruption as a mediator of brain trauma and chronic traumatic encephalopathy. Neurosci Biobehav Rev. 2018 Jan;84:316-324. doi: 10.1016/j.neubiorev.2017.08.016. Epub 2017 Aug 30. PMID: 28859995.
52. Aaron J. Schain,Agustin Melo-Carrillo, Andrew M. Strassman,Rami Burstein Cortical Spreading Depression Closes Paravascular Space and Impairs Glymphatic Flow: Implications for Migraine Headache. J Neurosci. 2017 Mar 15; 37(11): 2904–2915. doi: 10.1523/JNEUROSCI.3390-16.2017
53. Humm AM, Bostock H, Troller R, Z'Graggen WJ. Muscle ischaemia in patients with orthostatic hypotension assessed by velocity recovery cycles. J Neurol Neurosurg Psychiatry. 2011 Dec;82(12):1394-8. doi: 10.1136/jnnp-2011-300444. Epub 2011 Jun 7. PMID: 21653205.
54. Song J, da Costa KA, Fischer LM, et al. Polymorphism of the PEMT gene and susceptibility to nonalcoholic fatty liver disease (NAFLD). FASEB J. 2005;19(10):1266-1271. doi:10.1096/fj.04-3580com
55. Malengier-Devlies B, Filtjens J, Ahmadzadeh K, Boeckx B, Vandenhaute J, De Visscher A, Bernaerts E, Mitera T, Jacobs C, Vanderbeke L, Van Mol P, Van Herck Y, Hermans G, Meersseman P, Wilmer A, Gouwy M, Garg AD, Humblet-Baron S, De Smet F, Martinod K, Wauters E, Proost P, Wouters C, Leclercq G, Lambrechts D, Wauters J, Matthys P. Severe COVID-19 patients display hyper-activated NK cells and NK cell-platelet aggregates. Front Immunol. 2022 Oct 5;13:861251. doi: 10.3389/fimmu.2022.861251. PMID: 36275702; PMCID: PMC9581751.
56. Di Vito C, Calcaterra F, Coianiz N, Terzoli S, Voza A, Mikulak J, Della Bella S, Mavilio D. Natural Killer Cells in SARS-CoV-2 Infection: Pathophysiology and Therapeutic Implications. Front Immunol. 2022 Jun 30;13:888248. doi: 10.3389/fimmu.2022.888248. PMID: 35844604; PMCID: PMC9279859.
57. van Eeden C, Khan L, Osman MS, Cohen Tervaert JW. Natural Killer Cell Dysfunction and Its Role in COVID-19. Int J Mol Sci. 2020 Sep 1;21(17):6351. doi: 10.3390/ijms21176351. PMID: 32883007; PMCID: PMC7503862.
58. Dwivedi,R. Researchers study COVID-19 immune dysfunction in relation to phosphatidylserine. https://www.news-medical.net/news/20210623/Researchers-study-COVID-19-immune-dysfunction-in-relation-to-phosphatidylserine.aspx
59. Wikipedia. Catechol-O-methyltransferase .https://en.wikipedia.org/wiki/Catechol-O-methyltransferase
60. Zhu BT, Liehr JG. Inhibition of catechol O-methyltransferase-catalyzed O-methylation of 2- and 4-hydroxyestradiol by quercetin. Possible role in estradiol-induced tumorigenesis. J Biol Chem. 1996 Jan 19;271(3):1357-63. doi: 10.1074/jbc.271.3.1357. PMID: 8576124.
61. Tahara T, Shibata T, Arisawa T, Nakamura M, Yamashita H, Yoshioka D, Okubo M, Maruyama N, Kamano T, Kamiya Y, Fujita H, Nagasaka M, Iwata M, Takahama K, Watanabe M, Hirata I. Impact of catechol-O-methyltransferase (COMT) gene polymorphism on promoter methylation status in gastric mucosa. Anticancer Res. 2009 Jul;29(7):2857-61. PMID: 19596974
62. Van der Knaap LJ, Schaefer JM, Franken IH, Verhulst FC, van Oort FV, Riese H. Catechol-O-methyltransferase gene methylation and substance use in adolescents: the TRAILS study. Genes Brain Behav. 2014 Sep;13(7):618-25. doi: 10.1111/gbb.12147. Epub 2014 Jun 24. PMID: 24902721
63. Song J, da Costa KA, Fischer LM, et al. Polymorphism of the PEMT gene and susceptibility to nonalcoholic fatty liver disease (NAFLD). FASEB J. 2005;19(10):1266-1271. doi:10.1096/fj.04-3580com
64. Sumantri S, Rengganis I. Immunological dysfunction and mast cell activation syndrome in long COVID. Asia Pac Allergy. 2023 Mar;13(1):50-53. doi: 10.5415/apallergy.0000000000000022. Epub 2023 Apr 28. PMID: 37389095; PMCID: PMC10166245.
65. Eaton-Fitch N, Du Preez S, Cabanas H, Muraki K, Staines D, Marshall-Gradisnik S. Impaired TRPM3-dependent calcium influx and restoration using Naltrexone in natural killer cells of myalgic encephalomyelitis/chronic fatigue syndrome patients. J Transl Med. 2022 Feb 16;20(1):94. doi: 10.1186/s12967-022-03297-8. PMID: 35172836; PMCID: PMC8848670.
66. Marshall-Gradisnik et al. Examination of Single Nucleotide Polymorphisms (SNPs) in Transient Receptor Potential (TRP) Ion Channels in Chronic Fatigue Syndrome Patients. Immunology and Immunogenetics Insights 2015:7 1–6 doi:10.4137/III.S25147.
67. Barnden L, Thapaliya K, Eaton-Fitch N, Barth M, Marshall-Gradisnik S. Altered brain connectivity in Long Covid during cognitive exertion: a pilot study. Front Neurosci. 2023 Jun 22;17:1182607. doi: 10.3389/fnins.2023.1182607. PMID: 37425014; PMCID: PMC10323677.
68. Kaltoft,M et al. Lipoprotein(a) during COVID-19 hospitalization: Thrombosis,inflammation, and mortality. 2022. Atherosclerosis https://doi.org/10.1016/j.atherosclerosis.2022.07.015
69. Mahley RW. Apolipoprotein E: from cardiovascular disease to neurodegenerative disorders. J Mol Med (Berl). 2016 Jul;94(7):739-46. doi: 10.1007/s00109-016-1427-y. Epub 2016 Jun 9. PMID: 27277824; PMCID: PMC4921111.
70. Tindle, R. Long COVID: Sufferers can take heart. AJGP. 2024. doi: 10.31128/AJGP-07-23-6896
71. Couzin-Frankel J, Vogel G. Vaccines may cause rare, long COVID-like symptoms. Science 2022;375(6579):364–66. doi: 10.1126/science.ada0536.
72. Hulscher N, Procter BC, Wynn C, McCullough PA. Clinical approach to post-acute sequelae after COVID-19 infection and vaccination. Cureus. 2023;15(11):e49204. doi: 10.7759/cureus.49204.
73. Kwan AC, Ebinger JE, Wei J, et al. Apparent risks of postural orthostatic tachycardia syndrome diagnoses after COVID-19 vaccination and SARS-Cov-2 infection. Nat Cardiovasc Res 2022;1(12):1187–94. doi: 10.1038/s44161-022-00177-8
74. Rosner CM, Genovese L, Tehrani BN, et al. Myocarditis temporally associated with COVID-19 vaccination. Circulation 2021;144(6):502–505. doi: 10.1161/CIRCULATIONAHA.121.055891.
75. Krumholz HM, Wu Y, Sawano M, et al. Post-vaccination syndrome: A descriptive analysis of reported symptoms and patient experiences after Covid-19 Immunization. medRxiv [Preprint] 2023:2023.11.09.23298266. doi: 10.1101/2023.11.09.23298266.
76. Okuno S, Higo S, Kondo T, et al. SARS-CoV-2 spike receptor-binding domain is internalized and promotes protein ISGylation in human induced pluripotent stem cell-derived cardiomyocytes. Sci Rep 2023;13(1):21397. doi: 10.1038/s41598-023-48084-7.
77. Uversky VN, Redwan EM, Makis W, Rubio-Casillas A. IgG4 antibodies induced by repeated vaccination may generate immune tolerance to the SARS-CoV-2 spike protein. Vaccines (Basel) 2023;11(5):991. doi: 10.3390/vaccines11050991
78. Joseph P, Singh I, Oliveira R, Capone CA, Mullen MP, Cook DB, Stovall MC, Squires J, Madsen K, Waxman AB, Systrom DM. Exercise Pathophysiology in Myalgic Encephalomyelitis/Chronic Fatigue Syndrome and Postacute Sequelae of SARS-CoV-2: More in Common Than Not? Chest. 2023 Sep;164(3):717-726. doi: 10.1016/j.chest.2023.03.049. Epub 2023 Apr 11. PMID: 37054777; PMCID: PMC10088277.
79. Katz DL, Greene L, Ali A, Faridi Z. The pain of fibromyalgia syndrome is due to muscle hypoperfusion induced by regional vasomotor dysregulation. Med Hypotheses. 2007;69(3):517-25. doi: 10.1016/j.mehy.2005.10.037. Epub 2007 Mar 21. PMID: 17376601.
80. Wirth, K.J., Scheibenbogen, C. & Paul, F. An attempt to explain the neurological symptoms of Myalgic Encephalomyelitis/Chronic Fatigue Syndrome. J Transl Med 19, 471 (2021). https://doi.org/10.1186/s12967-021-03143-3
81. Bragée B, Michos A, Drum B, Fahlgren M, Szulkin R, Bertilson BC. Signs of Intracranial Hypertension, Hypermobility, and Craniocervical Obstructions in Patients With Myalgic Encephalomyelitis/Chronic Fatigue Syndrome. Front Neurol. 2020 Aug 28;11:828. doi: 10.3389/fneur.2020.00828. PMID: 32982905; PMCID: PMC7485557.
82. Hulens M, Dankaerts W, Rasschaert R, Bruyninckx F, De Mulder P, Bervoets C. The Link Between Empty Sella Syndrome, Fibromyalgia, and Chronic Fatigue Syndrome: The Role of Increased Cerebrospinal Fluid Pressure. J Pain Res. 2023 Jan 25;16:205-219. doi: 10.2147/JPR.S394321. PMID: 36721849; PMCID: PMC9884441.
83. Appelman, B., Charlton, B.T., Goulding, R.P. et al. Muscle abnormalities worsen after post-exertional malaise in long COVID. Nat Commun 15, 17 (2024). https://doi.org/10.1038/s41467-023-44432-3
84. Germain A, Giloteaux L, Moore GE, Levine SM, Chia JK, Keller BA, Stevens J, Franconi CJ, Mao X, Shungu DC, Grimson A, Hanson MR. Plasma metabolomics reveals disrupted response and recovery following maximal exercise in myalgic encephalomyelitis/chronic fatigue syndrome. JCI Insight. 2022 May 9;7(9):e157621. doi: 10.1172/jci.insight.157621. PMID: 35358096; PMCID: PMC9090259.
85. Boomershine,C. Fibromyalgia. 2023. Medscape. https://emedicine.medscape.com/article/329838-overview#a1
86. Coss-Adame, E., Rao, S. Brain and Gut Interactions in Irritable Bowel Syndrome: New Paradigms and New Understandings Curr Gastroenterol Rep. 2014 April ; 16(4): 379. doi:10.1007/s11894-014-0379-z.
87. Bashashati,M.,Moradi, M., Sarosiek,I.: Interleukin-6 in irritable bowel syndrome: A systematic review and meta-analysis of IL-6 (-G174C) and circulating IL-6 levels. Cytokine. 2017 Nov;99:132-138. doi: 10.1016/j.cyto.2017.08.017. Epub 2017 Sep 5. https://www.ncbi.nlm.nih.gov/pubmed/28886490
88. Wood PB, Schweinhardt P, Jaeger E, Dagher A, Hakyemez H, Rabiner EA, Bushnell MC, Chizh BA. Fibromyalgia patients show an abnormal dopamine response to pain. Eur J Neurosci. 2007 Jun;25(12):3576-82. doi: 10.1111/j.1460-9568.2007.05623.x. PMID: 17610577.
89. Becker S, Schweinhardt P. Dysfunctional neurotransmitter systems in fibromyalgia, their role in central stress circuitry and pharmacological actions on these systems. Pain Res Treat. 2012;2012:741746. doi: 10.1155/2012/741746. Epub 2011 Oct 2. PMID: 22110944; PMCID: PMC3195783.
90. Zheng, M., Karki, R., Williams, E.P. et al. TLR2 senses the SARS-CoV-2 envelope protein to produce inflammatory cytokines. Nat Immunol 22, 829–838 (2021). https://doi.org/10.1038/s41590-021-00937-x
Comments