Dr Graham Exelby September 2024
In the complex pathophysiology that lies beneath POTS, Fibromyalgia and their comorbidities, persistent mould exposure, sustained stress and PTSD stand out in many patients. These activate and dysregulate the immune response, activating NFKB with potential consequences. These can also complicate problems such as Long COVID, just as COVID can disrupt the neurotransmitters causing psychological symptoms.
The interplay between PTSD, chronic stress, and mould exposure can create a perfect storm for immune dysregulation and potential autoimmune activation. TLR4 signalling appears to be a central player in this process, serving as a link between environmental stressors and the immune response. Dysfunctional TLR4 signalling, resulting from chronic activation, may fail to resolve inflammation, leading to a sustained inflammatory state that can potentially break immune tolerance and contribute to autoimmune processes.
The geographical distribution of the POTS, Long COVID and Fibromyalgia patients seen in our clinic, appears to show a disproportionate number in recent years from the Northern Rivers region of NSW where there have been serious floodings, leaving occupants with both continuing mould exposure, sustained stress and PTSD, potentially dysregulating the immune system, with mould in particular activating the TLR2/ NF-κB pathway.
The pathophysiology of COVID-19 is characterized by systemic inflammation, hypoxia resulting from respiratory failure, and neuroinflammation (either due to viral neurotropism, the ability of the virus to invade and live in neural tissue, or in response to the cytokine storm), affecting the brain. (1)
The brain and spinal cord, which make up the CNS, are not usually accessed directly by pathogenic factors in the body's circulation due to a series of endothelial cells (single layer of squamous cells that form an interface between circulating blood or lymph in the vessels, controlling the flow of substances into and out of a tissue) known as the blood -brain barrier (BBB) (formed from endothelial cells, astrocyte end-feet and pericytes.)
The BBB prevents most infections from reaching the vulnerable nervous tissue. In the case where infectious agents are directly introduced to the brain or cross the blood–brain barrier, microglial cells must react quickly to decrease inflammation and destroy the infectious agents before they damage the sensitive neural tissue, so basically are the “first responders” in infections.
Figure 1: TLR Signalling in innate immune cells

Source: Duan T, Du Y, Xing C, Wang HY, Wang RF. Toll-Like Receptor Signaling and Its Role in Cell-Mediated Immunity. Front Immunol. 2022 Mar 3;13:812774. doi: 10.3389/fimmu.2022.812774. PMID: 35309296; PMCID: PMC8927970. (2)
Toll-Like Receptors
To understand what is happening we start with the immune response to COVID as described in Long COVID Immune Dysfunction (3)
In humans there are 10 types of body threat receptors, or Toll-Like Receptors (TLRs) that respond to a variety of PAMPs (pathogen-associated molecular patterns associated with bacteria and viruses). TLRs are crucial components in the initiation of the innate immune system, triggering the downstream production of pro-inflammatory cytokines, interferons (IFNs) and other mediators.
TLRs recognize invading pathogens by sensing PAMP and activate the regulation of innate immunity and cytokines. TLR activation leads to the production of proinflammatory cytokines and Interferon IFN.(4) TLRs 1,2,4,5,6,10 are plasma protein TLRs, while TLR3 and 7 and TLR9 are on endosomes (intracellular sorting organelles). TLR2/6 and TLR4 are located on the cell membrane.(Figure 2)
TLR4 signalling in COVID is activated by the Spike protein (S). This can lead to a pro-thrombotic and pro-inflammatory state contributing to severe complications eg myocardial infarction and acute lung injury.(5)(6)
Toll-like receptors TLR5, TLR4 and the heterodimers of TLR2-TLR1 or TLR2-TLR6 prefer to recognize the membrane components of pathogens on the cell surface. TLR3, TLR7-TLR8 and TLR9 localize to the endosome where they recognize nucleic acids from both the host and pathogens. TLR4 localizes at the plasma membrane but it is endocytosed into endosomes upon activation. The TLR signalling switches from MyD88 to TRIF once TLR4 moves to the endosomes.(38)
TLR2 senses the SARS-CoV-2 envelope protein (E), resulting in production of inflammatory cytokines and chemokines, contributing to the hyperinflammatory state and tissue damage seen in severe Covid. The severity of the Covid infection is thought to be largely determined by the E Protein /TLR2 activation rather than the S protein.(4)(7)
The endosomal TLR3 senses intracellular viral dsRNA. Activated TLR regulates the production of proinflammatory factors through a series of signalling in the NF‐κB pathway and activates IRF3/7 to produce I IFN. (4)(8) A DNA variant in TLR3 has also been identified as increasing susceptibility and mortality to acute COVID infections by decreasing TLR3 expression and impairing recognition of SARS-Co-V dsRNA. (4) These results suggest perivascular inflammation may be a critical factor in Long COVID, but the role of these receptors in Long COVID associated POTS has not been fully established.
TLR9 activation helps limit excessive inflammatory responses following traumatic experiences.(9) The failure of TLR9 to limit inflammation appears to play a significant role in post-traumatic stress disorder (PTSD) and associated anxiety as it serves as a crucial protective mechanism against post-traumatic inflammation.(9) Genetic polymorphisms in the TLR9 gene may predispose individuals to TLR9 dysfunction which could potentially affect its expression or function, altering the innate immune response and increasing susceptibility to post-traumatic inflammation and anxiety.(9)
Linking COVID, mould, PTSD and chronic stress
There are several connections between PTSD, chronic stress, mould exposure, and autoimmune activation, with TLR4 signalling and mast cell dysregulation playing a pivotal role in each. TLR4 signalling serves as a link between environmental stressors and the immune response. Dysfunctional TLR4 signalling, resulting from chronic activation, may fail to resolve inflammation, leading to a sustained inflammatory state that can potentially break immune tolerance and contribute to autoimmune processes. Dysfunctional TLR4 activation and an exaggerated cytokine storm typifies the immune response to the COVID spike protein.
Mast cell dysregulation: Mast cells, microglia, and astrocytes play important roles both independently and collectively in mediating responses to sustained mould exposure, stress and PTSD. Mast cells regulate the functions of immune cells such as dendritic cells, monocytes/macrophages, granulocytes, T cells, B cells and Natural Killer (NK) cells.
Mast cells act as "first responders" in stress conditions, rapidly degranulating and releasing pre-formed mediators. Chronic stress and PTSD can dysregulate mast cells by increased mast cell activation and degranulation due to elevated stress hormones like cortisol and noradrenalin, with enhanced production of pro-inflammatory mediators by mast cells, including histamine, cytokines, and chemokines, playing a crucial role in both peripheral inflammation and neuroinflammation in PTSD.(46)
Mould can impact on mast cells through direct activation of mast cells by mould spores and mycotoxins with increased production of inflammatory mediators like histamine and leukotrienes.(47)
Sensitization of mast cells can lead to heightened reactivity to other triggers.(47)
Activation of mast cells in the brain triggers activation of microglia, increased cytokine and TLR4 expression.(43)
Mast cells recruit immune cells to inflamed tissue by secreting chemokines and other mediators which locally increase vascular permeability.
Histamine and tryptase can degrade the extracellular matrix and disrupt the integrity of connective tissue.(40)(41)
Proteases eg chymase can inhibit collagen synthesis by smooth muscle cells, weakening the connective tissue structure, and mast cell-derived mediators like TNFa can induce apoptosis of smooth muscle cells, further compromising the connective tissue. Prostaglandins and leukotrienes contribute to inflammation and pain.(40)(41)
Neuroexcitatory glutamate binds to glutamate receptors on the mast cell surface. Glutamate has extensive effects on gene expression in the mast cells, including the upregulation of pro-inflammatory components such as IL-6 and CCL2.(42)
TLR4 Signalling and Inflammation: TLR4 is a key pattern recognition receptor that can be activated by both pathogen-associated molecular patterns (PAMPs) and damage-associated molecular patterns (DAMPs). (52) In the context of PTSD, chronic stress, and mould exposure:
Chronic stress can lead to the release of endogenous DAMPs that activate TLR4.(10)
Mould components, particularly mycotoxins, can act as PAMPs and activate TLR4.(11)
TLR4 activation triggers inflammatory signalling cascades, including the NF-κB pathway, leading to the production of pro-inflammatory cytokines.(10)
Dysfunctional TLR4 Signalling: In the context of chronic stress and mould exposure, TLR4 signalling may become dysfunctional, leading to exaggerated or prolonged inflammatory responses.(10)
This dysfunctional signalling can result in a failure to resolve inflammation, potentially contributing to autoimmune activation.(12)
The NLRP3 inflammasome, which is primed by TLR4 activation, may play a role in sustaining inflammation and potentially contributing to autoimmune processes.(10) Inflammasomes are large cytosolic multiprotein complexes that assemble in response to detection of infection- or stress-associated stimuli and lead to the activation of caspase-1-mediated inflammatory responses, including cleavage and unconventional secretion of the leaderless proinflammatory cytokines IL-1β and IL-18, and initiation of an inflammatory form of cell death referred to as pyroptosis. (13)
Chronic Stress and metabolic dysfunction, HPA axis and Immune Dysregulation:
Chronic stress and PTSD are linked to hypothalamic-pituitary-adrenal (HPA) axis dysfunction, which can lead to glucocorticoid resistance and impaired regulation of inflammation.(14) This HPA axis dysregulation may interfere with TLR9's normal immunomodulatory functions, contributing to unchecked inflammation.(9)
TLR9 dysfunction can have several consequences in metabolic disorders, increased inflammation in adipose tissue, leading to more severe metabolic disorders, weight gain and increased body fat, increased severity of glucose intolerance and insulin resistance.(15)
TLR9 dysfunction can result in altered immune cell populations and elevated proinflammatory cytokines and chemokines.(15)
TLR9 deficiency was associated with altered gut microbial communities, including reduced Lachnospiraceae, which may contribute to metabolic dysregulation.(16)
TLR9 plays a role in regulating inflammation and lipid metabolism in the liver, and its dysfunction may contribute to non-alcoholic fatty liver disease.(17)
Chronic stress, as seen in PTSD, can alter the balance between Type 1 and Type 2 cytokines.(18)
Stress hormones like glucocorticoids can suppress or dysregulate immune responses.(19)
This dysregulation can create a low-grade inflammatory state, potentially contributing to autoimmune activation.(19)
5. Mould Exposure and Immune Activation:
Mould exposure can trigger the Cell Danger Response (CDR), activating innate immune responses.(51)
Mycotoxins from mould can induce oxidative stress and mitochondrial dysfunction, further contributing to immune activation.(51)
6. Autoimune Activation Pathways:
The persistent activation of TLR4 by chronic stress and mould exposure can lead to sustained inflammation.(11)
This chronic inflammation can potentially break immune tolerance and contribute to autoimmune responses.(14)
The interplay between mast cells, microglia, and astrocytes, which all express TLR4, can amplify and sustain neuroinflammation, potentially contributing to autoimmune processes in the central nervous system.
7. Gut-Brain Axis and Autoimmunity: this pathway is seen in COVID with disruption of the gut-blood barrier, the tight junctions between epithelial cells increasing intestinal permeability leading to a diagnosis of “leaky gut.” (20)(21)
Like COVID, chronic stress and mould exposure can affect the gut microbiota, potentially leading to increased intestinal permeability.(11)(18)
The compromised gut barrier allows increased translocation of bacteria, bacterial products, and endotoxins from the intestinal lumen into the bloodstream, further activating TLR4 and potentially contributing to systemic inflammation and autoimmune responses.(18)
Depletion of beneficial commensal bacteria and enrichment of opportunistic pathogens is observed in COVID, (22)(23) with increased activation of mucosal CD4+ and CD8+ T cells, neutrophils, dendritic cells, and macrophages and a decrease in regulatory T cells, promoting an overactivated immune response.(20)
In mould exposure, mycotoxins produced by mould can disrupt the balance of beneficial and pathogenic bacteria in the gut, leading to dysbiosis and reduced microbial diversity in the gut, and can impair the gut microbiota's ability to bind and detoxify mycotoxins
The gut inflammatory response is characterized by increased production of various cytokines and chemokines with elevated levels of pro-inflammatory cytokines like IL-6, IL-1β, and TNF-α and increased expression of type I and III interferons contributes to the antiviral response but can also promote inflammation.(20)(21)
NFkB activation
Nuclear Factor kappa B (NFkB) is a protein complex that plays a crucial role in regulating the immune response, inflammation, and cell survival. The primary function of NFkB is to control gene expression in response to various signals, such as pro-inflammatory cytokines, bacterial or viral products, stress, and oxidative damage. NF-κB has long been considered a prototypical proinflammatory signalling pathway, largely based on the activation of NF-κB by proinflammatory cytokines such as interleukin 1 (IL-1) and tumour necrosis factor α (TNFα), and the role of NF-κB in the expression of other proinflammatory genes.
Anilkumar and Wright-Jin (24) describe that under normal situations NF-kB is inactivated and sequestered to the cytoplasm either by natural inhibitors of NF-kB or inherent structural inactivation. Dysregulation of NF-kB signalling has been implicated in various health conditions, including processes of synaptic plasticity and memory, autoimmune disorders, inflammatory diseases, cancer, and neurodegenerative diseases. Acting as primary immune responders in the CNS, microglia upregulate NFkB and cross-talk with other cells in the CNS can induce cell death, exacerbating the disease pathology.(24) (Figure 3)
NF-kB plays a multi-faceted role in the CNS, with diverse roles depending on physiological conditions. NF-kB activation in neurons is essential for information processing and transmission with both neuroprotective and neurodegenerative effects, and a key modulator of neuron survival, and typically protective. NF-kB activation in microglia and astrocytes is primarily associated with inflammation secondary to pathology and typically has detrimental effects.(24)
Activation of NF-kB in astrocytes is multi-faceted mirroring the astrocyte function in the CNS. NF-kB is implicated in astrocyte-dependent clearing of synaptic glutamate, metabolic control and modulation of astrocyte structural plasticity. In disease, the astrocytic NF-κB signalling is predominantly associated with increased inflammation, and can be associated with adverse outcomes. (24)
Hypoxia-induced NF-κB signalling is a major mediator of microglial inflammation associated with upregulated TLR4 activation. NF-κB signalling is implicated in both amyloid beta plaques and tau fibrils in late-onset Alzheimer’s disease, each mediated in part by microglial activation.(24)
The dysregulation of the inflammatory response in COVID-19 plays a very important role in disease progression. It has been observed that abnormal activity of NF-κB is directly associated with increased production of proinflammatory factors.(25)
Dysregulation of NFkB signalling has been implicated in various conditions, including autoimmune disorders, inflammatory diseases, malignancy, and neurodegenerative diseases.(26)
Upon recognition, TLRs recruit the adaptor protein MyD88 which initiates a signalling cascade involving IRAK kinases and TRAF6, ultimately leading to the activation of the IKK complex.(27) (Figure 2)
This complex phosphorylates the inhibitory protein lκB causing degradation, releasing NF-κB inducing the transcription of pro-inflammatory genes and production of pro-inflammatory cytokines IL-1ß, IL-6, and TNF-a and other enzymes eg COX2 and adhesion molecules.(28)
Figure 2: Downstream Signalling Pathways of TLRs
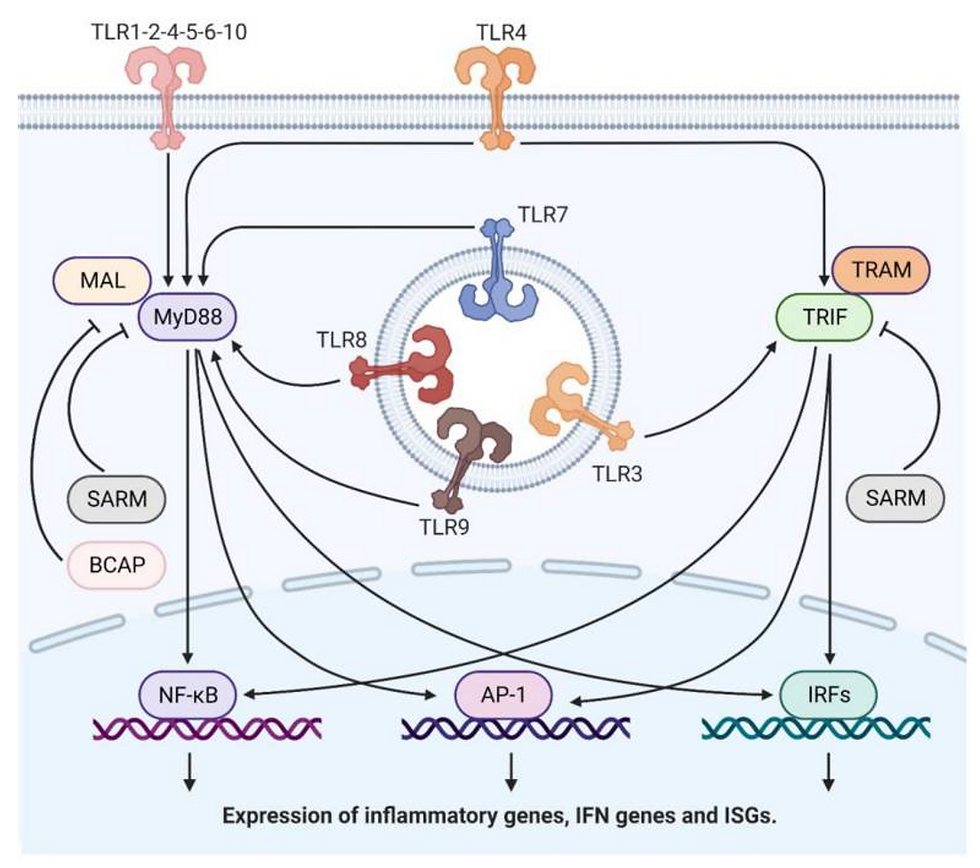
Source: Mantovani S, Oliviero B, Varchetta S, Renieri A, Mondelli MU. TLRs: Innate Immune Sentries against SARS-CoV-2 Infection. Int J Mol Sci. 2023 (4)
NF-κB -induced cytokine activation can cause local and systemic inflammation, as well as mast cell activation and its responses. Neurological complications can occur. The TLR2 and TLR4 receptor activation can affect the astrocyte /glutamate pathway, with its effect on sensitization and glymphatic function, as well as the TLR4/ microglial pathway with its effect on small fibre neuropathy. The TLR2/glutamate activation can upregulate MMP-9 and can compromise the BBB and increase neuroinflammation which can include seizures and chronic pain.
The Cellular Players:
Mast cells, microglia, and astrocytes play important roles both independently and collectively in mediating sustained stress responses in the central nervous system. The three cell types engage in complex crosstalk that can amplify neuroinflammatory responses.
Microglia:
Microglia are a type of neuroglia (glial cell in the CNS that do not produce electrical signals), that account for about 10-15% of cells found within the brain. Microglia are key cells in overall brain maintenance and constantly monitor neuronal functions.
Microglia scan the tissue and modify their morphology and functions if and when necessary. They are crucial for the formation, shaping, and functioning of synapses, fundamental for brain development during pre- and post-natal periods. (29)
Clough et al (30) describe: “Microglia are the resident immune cells of the Central Nervous System (CNS). Microglia have the capacity to migrate, proliferate and phagocytize. Under physiological conditions, microglia exist in their “resting” state, however on exposure to a pathogen, microglia transition into an activated state and quickly mobilize to the site of injury to initiate an innate immune response.” As the resident macrophage cells, they act as the first and main form of active immune defence in the CNS.
Damage to the brain triggers a specific type of reactive response mounted by neuroglia cells, in particular by microglia, the most prominent immune cells in the CNS and which are the first to respond to threat.(1) Inflammatory microglial activation (IL-6 and TNFa) is the most common brain pathology found in patients who died of COVID-19: 42% are affected, and another 15% have microclots in brain tissue.(31)
This is complicated by astrocyte/ microglial “cross-talk” and neurotransmitter dysregulation.(Figure 3) The SARS-Co-V spike protein activates microglia leading to pro-inflammatory effects and microglial-mediated synapse elimination. This microglial activation and neuroinflammation can disrupt the BBB. COVID also reduces the morphology and distribution of microglia and astrocytes in the hippocampus which has a major role in learning and memory. Mast cells promote cross-talk between T cells and myeloid cells like microglia during neuroinflammation, and the complex interplay between the activated microglia, reactive astrocytes and mast cells is a key part of the neurological manifestations of the COVID-19 infection.(19)(31)(32)
The complex nature of the immune response and mast cell activation in now an integral part of Long Covid pathogenesis. The same microglial activation has been demonstrated in other conditions -ME/CFS, ADHD, migraine, Fibromyalgia syndrome and Endometriosis.
Astrocytes
Astrocytes are the most abundant glial cells in the CNS. They are pivotal in maintaining CNS homeostasis, including neurotransmitter regulation, particularly glutamate. It is believed that astrocyte reactivity and subsequent glutamate dysregulation contributes to neurological symptoms eg cognitive impairment, fatigue and mood disorders in COVID, very similar to the dysfunction that occurred in the Gulf War Syndrome. If the brain is not directly damaged, resolution of systemic pathology usually results in restoration of the physiological homeostatic status of neuroglial cells. (1)
Astrocytes provide support for neurons and regulate the blood-brain barrier:
They become reactive under stress conditions
Reactive astrocytes release inflammatory mediators
They can impair blood-brain barrier integrity
Astrocytes modulate synaptic function and neurotransmission
Activation of TLR2 appears to affect the astrocytes. The “endfeet” of the astrocytes form the paravascular spaces thus dysfunction in the astrocytes can affect the glymphatic system function (reducing toxin clearance from the brain, and pressure modulation between the CSF and dural venous sinuses.) -The Glymphatic System (74) It is proposed that cerebrospinal fluid enters the brain via paravascular spaces along arteries, mixes with interstitial fluid, and leaves via paravascular spaces along veins.(33)
SARS-CoV-2 preferentially infects and replicates and propagates in astrocytes, particularly those adjacent to infected vasculature. In contrast, neurons and microglia are less likely to be directly infected. Importantly, while microglia and astrocytes are both reactivated, a direct dosage-sensitive effect of SARS-CoV-2 is only observed in reactive astrocytes. Astrocytes are the primary targets of SARS-CoV-2 in the brain. SARS-Co-V preferentially infects astrocytes over neurons resulting in astrocyte reactivation and neuronal death (31).
Blood flow in the brain is regulated by neurons and astrocytes. Attwell et al (34) describe “It is now recognized that neurotransmitter-mediated signalling has a key role in regulating cerebral blood flow, that much of this control is mediated by astrocytes, that oxygen modulates blood flow regulation, and that blood flow may be controlled by capillaries as well as by arterioles.” Astrocytes can promote the induction and progression of inflammatory states, which are significantly associated with the disease status or severity.(35)
The astrocyte/glutamate dysfunction has been found in the Gulf War Syndrome, where service personnel were plagued by a variety of medical problems including “neurodivergence,” caused by exposure to a number of toxic chemicals. This glutamate association has also been seen in Fibromyalgia, ADHD, Autism Spectrum, migraine, visual snow, Alzheimers and Parkinson’s Disease and other neurological dysfunction.
The mechanism proposed by Guedj and associates in Long Covid,(36) links these to astroglial/glutamate dysfunction. Astrocyte dysfunction, by affecting glymphatic function, is thought to play its role in fatigue and Intracranial Hypertension as “toxin” clearance in the brain and pressure modulation via the glymphatic system is impaired. Discussed in Amino Acid, Essential Vitamin and Mineral Burn off in Post Exertional Malaise (37)
Figure 3: Mast Cell, Microglia and Astrocyte Cross-Talk
The various histamine receptors have been described on each cell in this diagram, which may lead to a decision on where management may be directed.
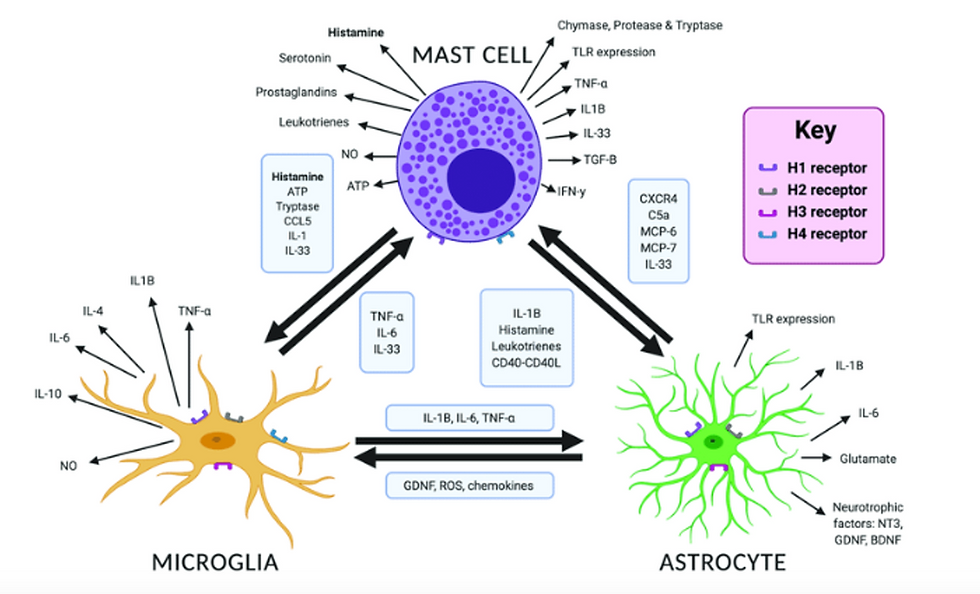
Source: Carthy, Elliott & Ellender, Tommas. (2021). Histamine, Neuroinflammation and Neurodevelopment: A Review. Frontiers in Neuroscience. 15. 10.3389/fnins.2021.680214.
Jin et al (38) describe the importance of a well-regulated immune response in minimising an immune insult while minimizing the risk of a dangerous uncontrolled pro-inflammatory reaction as seen in COVID infections. They showed that the cytokines mediated the activation of the vagal-brain axis, and dysregulation of the immune system and an enhanced inflammatory state linked to a broad range of diseases from diabetes to neurodegenerative disease.
Mast cells
The mast cell is a potent immune cell known for its functions in host defence responses and diseases, such as asthma and allergies. “Mast cells play a key role in homeostatic mechanisms and surveillance, recognizing and responding to different pathogens, and tissue injury. An abundance of mast cells reside in connective tissue that borders with the external world (the skin as well as gastrointestinal, respiratory, and urogenital tracts.) (39)
Mast cells are located perivascularly close to nerve endings and ANS sites eg carotid bodies and the adrenals, allowing them to potentially regulate and be affected by autonomic function. They can be triggered not only by allergens but also by triggers from the ANS, releasing neuro-sensitizing, pro-inflammatory and vasoactive mediators.
Mast cells regulate the functions of immune cells such as dendritic cells, monocytes/macrophages, granulocytes, T cells, B cells and Natural Killer (NK) cells. They recruit immune cells to inflamed tissue by secreting chemokines and other mediators which locally increase vascular permeability.
Mast cells are activated by cytokines from TLR4. They contribute to coronavirus-induced inflammation through mechanisms like degranulation and histamine release. Mast cell mediators can disrupt connective tissue integrity.
Histamine and tryptase can degrade the extracellular matrix and disrupt the integrity of connective tissue. Proteases eg chymase can inhibit collagen synthesis by smooth muscle cells, weakening the connective tissue structure, and mast cell-derived mediators like TNFa can induce apoptosis of smooth muscle cells, further compromising the connective tissue. Prostaglandins and leukotrienes contribute to inflammation and pain.(40)(41) Clinic observations have demonstrated collagen changes occurring after COVID infections.
Mast cells are increasingly seen as important in the communication between peripheral nerve endings and cells of the immune system. Alim et al (42) confirmed the binding of glutamate to glutamate receptors on the mast cell surface. Further, glutamate had extensive effects on gene expression in the mast cells, including the upregulation of pro-inflammatory components such as IL-6 and CCL2.(42)
Dong et al (43) demonstrated that brain inflammation plays a critical role in the pathophysiology of brain diseases. They demonstrated that in the brain, activation of mast cells triggers activation of microglia, increased cytokine and TLR4 expression, whereas stabilisation of mast cells with disodium cromoglycate (Cromolyn) inhibited the CNS inflammation that would otherwise result from activation of microglia.(43)
The prevalence of Mast Cell Activation Syndrome (MCAS) is similar to that of severe cases in the COVID infected population, and much of the COVID hyperinflammation is remarkably similar to mast cell-driven inflammatory processes. The wide range of symptoms seen in post-COVID conditions are those seen in POTS and its auto-immune co-morbidities, as described by Afrin et al(44) Malone et al (45) describe histamine exerting its biological actions through four types of histamine receptors (i.e., H1 receptor, H2 receptor, H3 receptor, and H4 receptor). It also activates acute immune-mediated reactions and enhances vascular smooth muscle contraction and the migration of other immune cells, antibodies, and mediators to the site of insult. The release of histamine by perivascular mast cells may also affect adjacent lymphatic vessel function inducing immune cell trafficking through its lumen, which potentially contribute to acute inflammatory stimulus.
Afrin (44) describes “Fatigue and malaise are the most common complaints in MCAS. Most patients remain functional, but some are severely impaired. Low-grade temperature dysregulation is not uncommon, as are lymph node swelling, weight loss, unexplained weight gain, loss of appetite, fluctuating oedema, but it is the gain in adipose tissue that accounts for weight increase in most MCAS. These patients may have bariatric surgery sometimes with complications of poor wound healing, and while there is initial weight loss, the other symptoms usually remain, and the weight gain slowly starts to return. Mast cells are programmed to site themselves at environmental interfaces- lungs, gut, skin, bladder, nose and sinuses etc, so there can be a wide range of pathology in aberrant mast cell activation.”
“Mast cell activation syndrome is known to permanently escalate its baseline level of dysfunction of the affected mast cells shortly after a major stressor, likely due to complex interactions between epigenetic abnormalities and the stressor’s induced cytokine storm- of additional mutations by the mutated stem cells from which the mutated /dysfunctional mast cells are derived.”(44)
The interplay between chronic stress, PTSD, mould exposure, and mast cell dysfunction creates a complex cycle that can significantly impact overall health and immune function. Addressing the root causes and supporting mast cell stabilization may be key to improving symptoms and immune regulation in affected individuals.
Chronic stress and PTSD can lead to dysregulation of mast cells in several ways:
Increased mast cell activation and degranulation due to elevated stress hormones like cortisol and noradrenalin, with enhanced production of pro-inflammatory mediators by mast cells, including histamine, cytokines, and chemokines. (46)
Lowered activation threshold of mast cells, making them more sensitive to triggers.(46)
Increased numbers of mast cells in stress-responsive areas of the brain and body.(46)
Mould can impact on mast cells through:
Direct activation of mast cells by mould spores and mycotoxins with increased production of inflammatory mediators like histamine and leukotrienes. (47)
Sensitization of mast cells, leading to heightened reactivity to other triggers.(47)
The combined effects on mast cells from chronic stress, PTSD, and mould exposure can lead to broader immune system dysfunction with dysregulation of T-cell responses and increased autoimmune reactivity, manifest as Mast Cell Activation Syndrome (MCAS), chronic fatigue, autoimmune disorder, multiple chemical sensitivities, GI tract dysfunction with compromised barrier function in the gut, lungs, and brain and cognitive impairment.
Natural Killer Cells
Manek and Singh (48) described: “A balanced immune regulation is crucial for recognizing an invading pathogen, its killing, and elimination. Toll‐like receptors (TLRs) are the key regulators of the innate immune system.”
Carter (49) describes: “The adaptive immune system includes the T cells and B cells. Unlike the cells of the innate immune system, T cells and B cells can identify specific features of pathogens – or cancer. DNA provides the instructions for a cell’s growth, survival and reproduction. When there’s a change in the DNA, it can cause a cell to divide more quickly and, in some cases, lead to cancer. It also slightly changes the protein the cell produces, but T cells and B cells can recognize this subtle difference and identify the cell as harmful.”(84)
T cells protect us from infection. In our daily lives, we’re constantly exposed to pathogens, such as bacteria, viruses and fungi. Without T lymphocytes, also called T cells, every exposure could be life-threatening. Cytotoxic T cells can wipe out infected or cancerous cells. They also direct the immune response by helping B lymphocytes to eliminate invading pathogens.(49)
B cells create antibodies. B lymphocytes, also called B cells, create proteins called antibodies that bind to pathogens or to foreign substances, such as toxins, to neutralize them. For example, an antibody can bind to a virus, which prevents it from entering a normal cell and causing infection. B cells can also recruit other cells to help destroy an infected cell. (49)
Natural Killer Cells (NK cells) are a type of cytotoxic lymphocyte critical to the innate immune system, representing 5-20% of all circulating lymphocytes in humans. The role of NK cells is analogous to that of cytotoxic T cells of the adaptive immune response. NK cells provide rapid responses to virus-infected cells, stressed cells, tumour cells, and other intracellular pathogens based on signals from several activating and inhibitory receptors.(9) NK cells can distinguish malignant from healthy cells.(50)
Under normal conditions, the natural killer (NK) cells of innate immunity and T‐cell of adaptive immune response have the tendency to cause the apoptosis of antigen presenting cells (APC) and prevent unnecessary activation and a balanced immune regulation is maintained, but any alterations in the lymphocyte catalytic activity due to the acquired infection in SARS‐COV‐2 infected patients dysregulate the immune balance by inactivating the NK cells as well cytotoxic T‐cells to kill the APC, leading to the dysregulated interactions of innate and adaptive immunity with consequent “cytokine storm.”(48)
Chronic stress associated with PTSD can lead to reductions in NK cell cytotoxic activity (NKCA). (51) While acute stress tends to increase NKCA, prolonged periods of stress, as seen in PTSD, can decrease it. The reductions in NKCA appear to be due to changes in the functional activity of NK cells, rather than changes in the numbers of circulating NK cells.(51) The stress response in PTSD, including alterations in the hypothalamic-pituitary-adrenal (HPA) axis and autonomic nervous system, can affect NK cell activity through neuroendocrine regulation.(52) PTSD appears to primarily decrease NK cell activity and function through chronic stress mechanisms, though the effects may vary based on factors like age and duration of stress exposure. This dysregulation of NK cells likely plays a role in the immune system dysfunction associated with PTSD.(53)
Mould exposure can lead to a decrease in NK cell numbers and cytotoxic activity against fungal pathogens. Chronic exposure to toxigenic moulds can result in abnormal NK cell activity, which may have neurological consequences.(54) Fungi can exert immunosuppressive effects on NK cells, potentially impairing their antifungal activity.(55)
Stress
Mast cells act as "first responders" in stress conditions.(56) They contain numerous secretory granules packed with pre-formed mediators, so upon activation, they rapidly degranulate and release these mediators which alerts and activates other immune cells, including microglia, the primary innate immune cells of the CNS. (56) They constantly survey the brain microenvironment Under stress, they become activated and change morphology. Activated microglia release pro-inflammatory mediators. They can phagocytose cellular debris and damaged neurons.(56)
Mast cells activate microglia and astrocytes through released mediators. Activated microglia and astrocytes further stimulate mast cells which creates feed-forward loops that sustain inflammation, with the interactions becoming exaggerated with aging and chronic stress.(57)
Chronic activation of these immune cells by sustained stress can lead to persistent neuroinflammation, blood-brain barrier disruption, neurotoxicity and neuronal death, and cognitive impairment and mood disorders. (57)
Morey et al (58) describe how the effect of stress on the immune system, while acute stress can temporarily enhance immune response, chronic or prolonged stress can have detrimental effects. Effective stress management strategies eg exercise, meditation and social support can mitigate the negative effects of stress on the immune system.
Chronic stress activates the sympathetic nervous system and the HPA axis. This releases inflammatory cytokines and increased systemic inflammation, contributing to various diseases and impaired immune responses. Dysregulation of the stress hormones, particularly nor-adrenaline can contribute to the hyperadrenergic state seen in many POTS.
Suppressed immune cell function – stress hormones eg cortisol can suppress the functions of vital cells eg Natural Killer Cells (NK cells), T cells, B cells, reducing their ability to effectively fight infections and other diseases.
Mast cells are well known for their role in allergic reactions. They are also involved in immune surveillance and modulation of the blood brain barrier. Sustained stress can lead to mast cell activation and degranulation, releasing histamine, cytokines, chemokines, collagenase, tryptase and other mediators. Mast cell overactivity can also increase vascular permeability causing fluid shifts that may contribute to orthostatic intolerance. Evolving research links mast cell overactivity and collagen dysfunction.
Mast cells are the first responders after trauma, which may include repetitive micro-trauma.
Dysregulation of TLR signalling, which can lead to an impaired immune response
Astrocytes play a crucial role in regulating immune responses in the central nervous system. The glutamatergic system is affected, with astrocytes exhibiting functional defects and impaired regulation of glutamate and glutamine homeostasis which may affect the neurological and cognitive symptoms. Glutamate is an excitatory neurotransmitter, and this function can be disrupted leading to excitotoxicity, neural sensitization and astrocyte dysfunction.
Stress can reactivate latent viruses eg EBV and HSV by suppressing the immune system’s ability to keep these viruses in a dormant state.
TLR4 plays a crucial role in stress-related inflammation and immune dysregulation.
TLR3 is an endogenous receptor, and recognizes double-stranded DNA. TLR3 activation in astrocytes can lead to production of inflammatory mediators, contributing to excitotoxicity and neuronal damage.
TLR7 and TLR9 recognize single-stranded RNA and unmethylated CpG DNA respectively, are implicated in the development of autoimmune diseases eg SLE and rheumatoid arthritis. Stress can exacerbate these conditions.
TLR2, which recognizes a variety of microbial components, has been associated with stress-related reactivation of latent viruses eg EBV and HSV. (58)
The interaction between stress and TLR signalling is bi-directional. Stress can influence TLR expression and function, and TLR activation can also affect the HPA axis and sympathetic nervous system, potentially exacerbating stress responses. This crosstalk can lead to a cycle of stress and inflammation that may contribute to the development of stress-related diseases.
Compounding this, as the immune cells of the brain, the microglia, astrocytes and mast cells are activated, there is cross talk between the 3 by cytokines production.(Figure 3)
PTSD:
Many individuals who coped at the time of their trauma become unwell at a later date. The majority of people who develop PTSD do not initially have acute stress disorders, although the majority of those who have an acute stress disorder are likely to develop subsequent PTSD.
Studies in military personnel have shown that a traumatic experience can lie relatively dormant only to become manifest at some future point. Sub-clinical symptoms leave the person at risk of progressive activation with environmental stress or trauma exposure (or from inflammatory cascades from threat receptor activation from genetic causes, spine and diet triggers.)
Delayed onset PTSD refers to cases where PTSD symptoms emerge 6 months or more after the traumatic event. This challenges earlier views that trauma symptoms always appear immediately and then gradually fade.(59) Individuals live in a dynamic environment in which traumatic events and other life stresses interact, with the progressive accumulation of risk. Multiple traumas can accumulate over time, increasing PTSD risk. Ongoing life stressors interact with trauma effects, and environmental triggers can reactivate trauma memories. (59)
Some key aspects of delayed onset PTSD include:
Symptoms can emerge months or even years after the initial trauma
It may involve a gradual worsening of subclinical symptoms over time
Prior trauma exposure can increase risk of delayed onset PTSD from subsequent traumas
Ongoing stress places strain on the adaptive capacity of individuals, leading to an increased risk of disease. The effects of stress on the hypothalamic pituitary adrenal axis and the autonomic nervous system have long been studied and the regulation of these systems is known as “allostatic load.” This refers to the wear and tear on the body in response to repeated cycles of stress. Not only is there a link to musculoskeletal symptoms but also an important risk for coronary artery disease.
Several factors contribute to the progressive accumulation of PTSD risk over time, with sensitization and kindling processes in the brain increase reactivity to stress, traumatic memories can be repeatedly triggered, reinforcing neural pathways. Avoidance behaviours may increase, limiting opportunities for processing trauma, and negative alterations in cognition and mood can worsen over time.
PTSD has long been seen as an “overlapping syndrome” with fibromyalgia, with significantly greater levels of avoidance, hyperarousal, re-experiencing, anxiety, and depression.(2) It is associated with dysregulation of the immune system, including activation of TLRs by DAMPs and alterations in TLR signalling pathways leading to neuroinflammation.
The glutamatergic system is affected, with astrocytes exhibiting functional defects and impaired regulation of glutamate and glutamine homeostasis which may affect the neurological and cognitive symptoms of the disorder. - Amino Acid, Essential Vitamin and Mineral Burn off in Post Exertional Malaise.(3)
TLR9 activation helps limit excessive inflammatory responses following traumatic experiences.(9) Katrinli et al (14) describe a failure of the innate immune system TLR9 to limit inflammation and involvement of NFκB-mediated enhancement of inflammatory reactions and the association of serum interleukin-1β that increases with symptoms severity and volumetric brain changes in post-traumatic stress disorder patients.(14)(9)
While TLR9 plays a protective role, other TLRs can have pro-inflammatory effects in PTSD. TLR4 is often associated with pro-inflammatory responses in PTSD, its activation leading to increased production of inflammatory cytokines. TLR2 may contribute to pro-inflammatory responses, though its role is less well-defined than TLR4. Some studies suggest TLR3 may have both pro- and anti-inflammatory effects.(14)
In addition to well-defined psychological and behavioural symptoms, PTSD also exhibit elevated concentrations of inflammatory markers, including C-reactive protein (CRP), interleukin-6 (IL-6), and tumour necrosis factor-α (TNF α.)
NF-κB activation plays an important role in the pathophysiology of PTSD, with increased NF-κB activation, associated with increased production of pro-inflammatory cytokines like IL-1β, TNF-α, and IL-6. The stress exposure increases NF-κB activation in brain regions like the hippocampus and prefrontal cortex.(64) NF-κB activation in the basolateral amygdala is required for fear memory reconsolidation, and inhibition of NF-κB in this region can disrupt the reconsolidation of fear memories.(60)
NF-κB activation promotes the transcription of genes involved in inflammation, mediates the production of pro-inflammatory cytokines, which are elevated in PTSD.(9)(14) There is also an interaction between NF-κB activation and the HPA axis. Cortisol, which is often dysregulated in PTSD, normally inhibits NF-κB signalling and reduces inflammatory cytokine production. Lowered cortisol levels in PTSD may lead to insufficient suppression of NF-κB-mediated inflammation.(61)
PTSD is often co-morbid with immune-related conditions, such as cardiometabolic and autoimmune disorders. Numerous factors, including lifetime trauma burden, biological sex, genetic background, metabolic conditions, and gut microbiota, may contribute to inflammation in PTSD. This increased inflammation can influence neural circuits and neurotransmitter signalling in regions of the brain relevant to fear, anxiety, and emotion regulation.(14)
There has an increasing recognition of a shared pattern of symptoms and aetiology between whiplash, fibromyalgia, IBS, chronic fatigue and PTSD. Disorders of the hypothalamic pituitary adrenal axis (HPA axis) have been identified in all these disorders (hence the current reference to “adrenal fatigue” by therapists trying to understand the links between these various problems). Such stress-induced changes have been associated with major impacts on neurogenesis and brain functioning, and studies suggest that the dysfunction of the HPA axis plays an important role in the onset of chronic widespread musculoskeletal pain.
Sensitization is a critical process in the onset of pain syndromes and PTSD. The central role of the amygdalae in the kindling in PTSD is similar to the phenomena of windup of C fibre evoked pain in fibromyalgia, IBS and chronic fatigue. Furthermore this has been associated with modified autonomic function (dysautonomia). The increasing evidence of brainstem hypoperfusion in these conditions is described in: Brainstem Hypoperfusion, Coat Hanger pain and Post-Exertional Malaise in POTS and Long COVID. (62)
Mast cells, microglia, and astrocytes play important roles both independently and collectively in post-traumatic stress disorder (PTSD). Mast cells act as "first responders" in stress conditions, rapidly degranulating and releasing pre-formed mediators. Mast cells play a crucial role in both peripheral inflammation and neuroinflammation in PTSD. (63)
They are among the first to respond to changes in the internal and external environment, secreting mediators within seconds to minutes upon stimulation. This alerts and activates other immune cells, including microglia. The synergistic interactions between mast cells, microglia, and astrocytes can amplify and sustain damaging neuroinflammation in PTSD.(63)(64)
Chronic activation of these immune cells in PTSD can lead to:
Persistent neuroinflammation (63)
Blood-brain barrier disruption (63)
Neurotoxicity and neuronal damage (63)
Cognitive impairment and behavioural changes (63)
This persistent inflammation may contribute to the chronic nature of PTSD symptoms and potentially increase the risk of developing neurodegenerative diseases.
The role of amino acid/neurotransmitter in sensitization, where immune overactivity triggers an overactive immune response involving mast cells, microglia and astrocytes is discussed in Amino Acid, Essential Vitamin and Mineral Burn off in Post Exertional Malaise (3)
PTSD it has been recognized that exposure to traumatic events leads to increased blood pressure, heart rate, and sympathetic activation of sweating in the hands, from increased activity of the sympathetic nervous system, and in particular, hyperfunction of the central noradrenergic system. Other studies have found a similar situation in hyperlipidaemia, obesity and metabolic syndrome, further increasing arteriosclerotic risk.
In essence, the internal physiological environment of a person adapts to external demands. When the body is repeatedly stressed the consequent allostatic state has the capacity to disrupt their health. Stress leads to a disruption of the glucocorticoid system, in concert with a range of other neuropeptides such as corticotrophin-releasing factor, beta endomorphin, neuropeptide Y and the catecholamines. The pattern of increasing sensitivity to environmental load can also become manifest as hypertension, hyperlipidaemia and obesity.(28) Targeting the interactions between these cell types, particularly mast cell activation, may offer therapeutic potential for mitigating the neurological effects of PTSD. However, more research is needed to fully elucidate the complex mechanisms involved in these cellular interactions in the context of PTSD.
Mould
Mast cells, microglia, and astrocytes play important roles both independently and collectively in mediating responses to sustained mould exposure in the central nervous system. Chronic activation of these immune cells by sustained mould exposure can lead to:
Persistent neuroinflammation (65)(66)
Blood-brain barrier disruption (67)
Neurotoxicity and neuronal damage (65)(66)
Cognitive impairment and behavioural changes (65)(66)(68)
For example, studies have shown that mould exposure can cause:
Hippocampal immune activation and decreased neurogenesis (65)
Deficits in learning, memory, and cognitive function (65)(68)
Alterations in pain sensitivity and anxiety-like behaviour. (65)
Mould can impact on mast cells through:
Direct activation of mast cells by mould spores and mycotoxins with increased production of inflammatory mediators like histamine and leukotrienes. (47)
Sensitization of mast cells, leading to heightened reactivity to other triggers.(47)
Mould is a common source of continuing inflammatory activation, through its activation of the NFkB pathway. It has been seen as a primary activator, or more commonly part of a series of injuries, parasites, stress, PTSD etc that together are responsible for the symptoms of POTS, Fibromyalgia and other conditions. Research has shown that the mould components are recognized by the TLRs both TLR2, TLR4 (and TLR6) activation is involved, (69)(70)
The immune response to mould exposure involves the activation of pattern recognition receptors (PRRs), particularly Toll-like receptors (TLRs) which recognize pathogen-associated molecular patterns (PAMPs) present in mould spores and cell wall components. This triggers a signalling cascade leading to activation of the transcription factor NFkB and subsequent production of pro-inflammatory cytokines and other inflammatory mediators.
Conclusion:
Mould, sustained stress and PTSD play important roles in immune system dysregulation. In POTS and Fibromyalgia these alone may be sufficient to activate and drive the inflammation and autonomic dysregulation. In Long COVID they play an important role in the persistence of symptoms. The inflammatory responses from these can be triggered into a feed-forward activation with persistence of symptoms and immune dysfunction.
Acting as primary immune responders in the CNS, microglia upregulate NFkB and cross-talk with other cells in the CNS can induce cell death, exacerbating the disease pathology. Dysregulation of NF-kB signalling has been implicated in various health conditions, including processes of synaptic plasticity and memory, autoimmune disorders, inflammatory diseases, cancer, and neurodegenerative diseases.
The interplay between chronic stress, PTSD, mould exposure, and mast cell dysfunction creates a complex cycle that can significantly impact overall health and immune function. Addressing the root causes and supporting mast cell stabilization may be key to improving symptoms and immune regulation in affected individuals.
References:
Phulwani NK, Esen N, Syed MM, Kielian T. TLR2 expression in astrocytes is induced by TNF-alpha- and NF-kappa B-dependent pathways. J Immunol. 2008 Sep 15;181(6):3841-9. doi: 10.4049/jimmunol.181.6.3841. PMID: 18768838; PMCID: PMC2649826
Duan T, Du Y, Xing C, Wang HY, Wang RF. Toll-Like Receptor Signaling and Its Role in Cell-Mediated Immunity. Front Immunol. 2022 Mar 3;13:812774. doi: 10.3389/fimmu.2022.812774. PMID: 35309296; PMCID: PMC8927970.
Exelby, G. Long COVID Immune Dysfunction. 2024. https://www.mcmc-research.com/post/long-covid-immune-dysfunction
Mantovani S, Oliviero B, Varchetta S, Renieri A, Mondelli MU. TLRs: Innate Immune Sentries against SARS-CoV-2 Infection. Int J Mol Sci. 2023 Apr 29;24(9):8065. doi: 10.3390/ijms24098065. PMID: 37175768; PMCID: PMC10178469
Aboudounya MM, Heads RJ. COVID-19 and Toll-Like Receptor 4 (TLR4): SARS-CoV-2 May Bind and Activate TLR4 to Increase ACE2 Expression, Facilitating Entry and Causing Hyperinflammation. Mediators Inflamm. 2021 Jan 14;2021:8874339. doi: 10.1155/2021/8874339. PMID: 33505220; PMCID: PMC7811571.
van der Donk LEH, Bermejo-Jambrina M, van Hamme JL, Volkers MMW, van Nuenen AC, Kootstra NA, Geijtenbeek TBH. SARS-CoV-2 suppresses TLR4-induced immunity by dendritic cells via C-type lectin receptor DC-SIGN. PLoS Pathog. 2023 Oct 16;19(10):e1011735. doi: 10.1371/journal.ppat.1011735. PMID: 37844099; PMCID: PMC10602378.
Sariol, A., Perlman, S. SARS-CoV-2 takes its Toll. Nat Immunol 22, 801–802 (2021). https://doi.org/10.1038/s41590-021-00962-w
Jiang, Yujie & Zhao, Tingmei & Zhou, Xueyan & Xiang, Yu & Gutierrez Castrellon, Pedro & Ma, Xuelei. (2022). Inflammatory pathways in COVID‐19: Mechanism and therapeutic interventions. MedComm. 3. 10.1002/mco2.154.
Zimmerman G, Shaltiel G, Barbash S, Cohen J, Gasho CJ, Shenhar-Tsarfaty S, Shalev H, Berliner SA, Shelef I, Shoham S, Friedman A, Cohen H, Soreq H. Post-traumatic anxiety associates with failure of the innate immune receptor TLR9 to evade the pro-inflammatory NFκB pathway. Transl Psychiatry. 2012 Feb 21;2(2):e78. doi: 10.1038/tp.2012.4. PMID: 22832815; PMCID: PMC3309554.
Souza-Junior FJC, Cunha LC, Lisboa SF. Toll-like receptor 4 in the interface between neuroimmune response and behavioral alterations caused by stress. Explor Neuroprot Ther. 2022;2:182–209. https://doi.org/10.37349/ent.2022.00028
Kondashevskaya, M.V., Artemyeva, K.A. & Mikhaleva, L.M. The Synergistic Trio of Metabolic Master Regulators Sustaining a Vicious Circle of Pathological Processes in Post-Traumatic Stress Disorder. J Evol Biochem Phys 60, 988–1002 (2024). https://doi.org/10.1134/S0022093024030116
Reino DC, Palange D, Feketeova E, Bonitz RP, Xu DZ, Lu Q, Sheth SU, Peña G, Ulloa L, De Maio A, Feinman R, Deitch EA. Activation of toll-like receptor 4 is necessary for trauma hemorrhagic shock-induced gut injury and polymorphonuclear neutrophil priming. Shock. 2012 Jul;38(1):107-14. doi: 10.1097/SHK.0b013e318257123a. PMID: 22575992; PMCID: PMC3378823.
de Zoete MR, Palm NW, Zhu S, Flavell RA. Inflammasomes. Cold Spring Harb Perspect Biol. 2014 Oct 16;6(12):a016287. doi: 10.1101/cshperspect.a016287. PMID: 25324215; PMCID: PMC4292152.
Katrinli, S., Oliveira, N.C.S., Felger, J.C. et al. The role of the immune system in posttraumatic stress disorder. Transl Psychiatry 12, 313 (2022). https://doi.org/10.1038/s41398-022-02094-7
Hong CP, Yun CH, Lee GW, Park A, Kim YM, Jang MH. TLR9 regulates adipose tissue inflammation and obesity-related metabolic disorders. Obesity (Silver Spring). 2015 Nov;23(11):2199-206. doi: 10.1002/oby.21215. Epub 2015 Aug 11. PMID: 26260403.
Wang P, Yang X, Zhang L, Sha S, Huang J, Peng J, Gu J, Pearson JA, Hu Y, Zhao H, Wong FS, Wang Q, Wen L. Tlr9 deficiency in B cells leads to obesity by promoting inflammation and gut dysbiosis. Nat Commun. 2024 May 18;15(1):4232. doi: 10.1038/s41467-024-48611-8. PMID: 38762479; PMCID: PMC11102548.
Nishimoto S, Fukuda D, Sata M. Emerging roles of Toll-like receptor 9 in cardiometabolic disorders. Inflamm Regen. 2020 Jul 21;40:18. doi: 10.1186/s41232-020-00118-7. PMID: 32714475; PMCID: PMC7374824.
Ingrosso DMF, Primavera M, Samvelyan S, Tagi VM, Chiarelli F. Stress and Diabetes Mellitus: Pathogenetic Mechanisms and Clinical Outcome. Horm Res Paediatr. 2023;96(1):34-43. doi: 10.1159/000522431. Epub 2022 Feb 4. PMID: 35124671.
Theoharides TC, Kempuraj D. Role of SARS-CoV-2 Spike-Protein-Induced Activation of Microglia and Mast Cells in the Pathogenesis of Neuro-COVID. Cells. 2023 Feb 22;12(5):688. doi: 10.3390/cells12050688. PMID: 36899824; PMCID: PMC10001285.
Eleftheriotis G, Tsounis EP, Aggeletopoulou I, Dousdampanis P, Triantos C, Mouzaki A, Marangos M, Assimakopoulos SF. Alterations in gut immunological barrier in SARS-CoV-2 infection and their prognostic potential. Front Immunol. 2023 Mar 15;14:1129190. doi: 10.3389/fimmu.2023.1129190. PMID: 37006316; PMCID: PMC10050566.
Sun, Z., Song, ZG., Liu, C. et al. Gut microbiome alterations and gut barrier dysfunction are associated with host immune homeostasis in COVID-19 patients. BMC Med 20, 24 (2022). https://doi.org/10.1186/s12916-021-02212-0
Zhang, F., Lau, R.I., Liu, Q. et al. Gut microbiota in COVID-19: key microbial changes, potential mechanisms and clinical applications. Nat Rev Gastroenterol Hepatol 20, 323–337 (2023). https://doi.org/10.1038/s41575-022-00698-4
Roy K, Agarwal S, Banerjee R, Paul MK, Purbey PK. COVID-19 and gut immunomodulation. World J Gastroenterol. 2021 Dec 14;27(46):7925-7942. doi: 10.3748/wjg.v27.i46.7925. PMID: 35046621; PMCID: PMC8678818.
Anilkumar S, Wright-Jin E. NF-κB as an Inducible Regulator of Inflammation in the Central Nervous System. Cells. 2024 Mar 11;13(6):485. doi: 10.3390/cells13060485. PMID: 38534329; PMCID: PMC10968931.
Gudowska-Sawczuk M, Mroczko B. The Role of Nuclear Factor Kappa B (NF-κB) in Development and Treatment of COVID-19: Review. Int J Mol Sci. 2022 May 9;23(9):5283. doi: 10.3390/ijms23095283. PMID: 35563673; PMCID: PMC9101079.
NF-κB. Wikipedia. https://en.wikipedia.org/wiki/NF-%CE%BAB
Kritas SK, Gallenga CE, D Ovidio C, Ronconi G, Caraffa Al, Toniato E, Lauritano D, Conti P. Impact of mold on mast cell-cytokine immune response. J Biol Regul Homeost Agents. 2018 Jul-Aug;32(4):763-768. PMID: 30043558.
Li, D., Wu, M. Pattern recognition receptors in health and diseases. Sig Transduct Target Ther 6, 291 (2021). https://doi.org/10.1038/s41392-021-00687-0
Steardo L Jr, Steardo L, Scuderi C. Astrocytes and the Psychiatric Sequelae of COVID-19: What We Learned from the Pandemic. Neurochem Res. 2023 Apr;48(4):1015-1025. doi: 10.1007/s11064-022-03709-7. Epub 2022 Aug 3. PMID: 35922744; PMCID: PMC9362636.
Clough E, Inigo J, Chandra D, Chaves L, Reynolds JL, Aalinkeel R, Schwartz SA, Khmaladze A, Mahajan SD. Mitochondrial Dynamics in SARS-COV2 Spike Protein Treated Human Microglia: Implications for Neuro-COVID. J Neuroimmune Pharmacol. 2021 Dec;16(4):770-784. doi: 10.1007/s11481-021-10015-6. Epub 2021 Oct 2. Erratum
Bayat AH, Azimi H, Hassani Moghaddam M, Ebrahimi V, Fathi M, Vakili K, Mahmoudiasl GR, Forouzesh M, Boroujeni ME, Nariman Z, Abbaszadeh HA, Aryan A, Aliaghaei A, Abdollahifar MA. COVID-19 causes neuronal degeneration and reduces neurogenesis in human hippocampus. Apoptosis. 2022 Dec;27(11-12):852-868. doi: 10.1007/s10495-022-01754-9. Epub 2022 Jul 25. PMID: 35876935; PMCID: PMC9310365.
Ransohoff RM, Brown MA. Innate immunity in the central nervous system. J Clin Invest. 2012 Apr;122(4):1164-71. doi: 10.1172/JCI58644. Epub 2012 Apr 2. PMID: 22466658; PMCID: PMC3314450.
Savelieff MG, Feldman EL, Stino AM. Neurological sequela and disruption of neuron-glia homeostasis in SARS-CoV-2 infection. Neurobiol Dis. 2022 Jun 15;168:105715. doi: 10.1016/j.nbd.2022.105715. Epub 2022 Mar 29. PMID: 35364273; PMCID: PMC8963977.
Attwell D, Buchan AM, Charpak S, Lauritzen M, Macvicar BA, Newman EA. Glial and neuronal control of brain blood flow. Nature. 2010 Nov 11;468(7321):232-43. doi: 10.1038/nature09613. PMID: 21068832; PMCID: PMC3206737.
Zhang,P et al. Enhanced Glial Reaction and Altered Neuronal Nitric Oxide Synthase are Implicated in Attention Deficit Hyperactivity Disorder. Front. Cell Dev. Biol, 2022. https://doi.org/10.3389/fcell.2022.901093
Hotowitz,T, Pellurin,L, Zimmer, E, Guedj,E. Brain fog in long COVID: A glutamatergic hypothesis with astrocyte dysfunction accounting for brain PET glucose hypometabolism. Elsevier, Medical Hypotheses. https://doi.org/10.1016/j.mehy.2023.111186
Exelby, G. Amino Acid, Essential Vitamin and Mineral Burn off in Post Exertional Malaise. 2024. https://www.mcmc-research.com/post/amino-acid-essential-vitamin-and-mineral-burn-off-in-post-exertional-malaise
Jin, S., Kim, J., Park, J. et al. Hypothalamic TLR2 triggers sickness behavior via a microglia-neuronal axis. Sci Rep 6, 29424 (2016). https://doi.org/10.1038/srep29424
Villapol S, Balarezo MG, Affram K, Saavedra JM, Symes AJ. Neurorestoration after traumatic brain injury through angiotensin II receptor blockage. Brain. 2015 Nov;138(Pt 11):3299-315. doi: 10.1093/brain/awv172. Epub 2015 Jun 26. PMID: 26115674; PMCID: PMC4731413.
Krystel-Whittemore M, Dileepan KN, Wood JG. Mast Cell: A Multi-Functional Master Cell. Front Immunol. 2016 Jan 6;6:620. doi: 10.3389/fimmu.2015.00620. PMID: 26779180; PMCID: PMC4701915
Beghdadi W, Madjene LC, Benhamou M, Charles N, Gautier G, Launay P, Blank U. Mast cells as cellular sensors in inflammation and immunity. Front Immunol. 2011 Sep 6;2:37. doi: 10.3389/fimmu.2011.00037. PMID: 22566827; PMCID: PMC3342044.
Alim MA, Grujic M, Ackerman PW, Kristiansson P, Eliasson P, Peterson M, Pejler G. Glutamate triggers the expression of functional ionotropic and metabotropic glutamate receptors in mast cells. Cell Mol Immunol. 2021 Oct;18(10):2383-2392. doi: 10.1038/s41423-020-0421-z. Epub 2020 Apr 20. Erratum in: Cell Mol Immunol. 2020 Sep 3;: PMID: 32313211; PMCID: PMC8484602.
Dong H, Zhang X, Wang Y, Zhou X, Qian Y, Zhang S. Suppression of Brain Mast Cells Degranulation Inhibits Microglial Activation and Central Nervous System Inflammation. Mol Neurobiol. 2017 Mar;54(2):997-1007. doi: 10.1007/s12035-016-9720-x. Epub 2016 Jan 21. PMID: 26797518
Afrin, Lawrence; Weinstock, Leonard; Molderings, Gerhard. Covid-19 Hyperinflammation and post-Covid 19 may be rooted in Mast Cell Activation Syndrome. 2020: International Journal of Infectious Diseases 100, 327-332.
Malone,R. et al. Covid-19: Famotidine,Histamine,Mast Cells and Mechanisms. 2021. Frontiers in Pharmacology, https://www.frontiersin.org/articles/10.3389/fphar.2021.633680/full
Tsai M, Grimbaldeston M, Galli SJ. MAST CELLS AND IMMUNOREGULATION/IMMUNOMODULATION. In: Madame Curie Bioscience Database [Internet]. Austin (TX): Landes Bioscience; 2000-2013. Available from: https://www.ncbi.nlm.nih.gov/books/NBK45995/
Mastocytosis and other Mast Cell Disorders. ASCIA. https://www.allergy.org.au/patients/allergy-testing/mastocytosis
Manik,M., Singh,R. Role of toll-like receptors in modulation of cytokine storm signalling in SARS_CoV-2-induced COVID-19. (2021) Journal of Medical Virology. DOI: 10.1002/jmv.27405
Carter, D. T Cells, B Cells and the Immune System. University of Texas MD Anderson Cancer Center. https://www.mdanderson.org/cancerwise/t-cells--b-cells-and-the-immune-system.h00-159465579.html
Waldhauer, I., Steinle, A. NK cells and cancer immunosurveillance. Oncogene 27, 5932–5943 (2008). https://doi.org/10.1038/onc.2008.267
Witek-Janusek L, Gabram S, Mathews HL. Psychologic stress, reduced NK cell activity, and cytokine dysregulation in women experiencing diagnostic breast biopsy. Psychoneuroendocrinology. 2007 Jan;32(1):22-35. doi: 10.1016/j.psyneuen.2006.09.011. Epub 2006 Nov 7. PMID: 17092654; PMCID: PMC3937868.
Capellino, S., Claus, M. & Watzl, C. Regulation of natural killer cell activity by glucocorticoids, serotonin, dopamine, and epinephrine. Cell Mol Immunol 17, 705–711 (2020). https://doi.org/10.1038/s41423-020-0477-9
Liberzon, Israel, and others, 'Inflammation and Immune Function in PTSD: Mechanisms, Consequences, and Translational Implications', in Israel Liberzon, and Kerry Ressler (eds), Neurobiology of PTSD: From Brain to Mind (New York, 2016; online edn, Oxford Academic, 1 Aug. 2016), https://doi.org/10.1093/med/9780190215422.003.0013, accessed 14 Sept. 2024.
Anyanwu E, Campbell AW, Jones J, Ehiri JE, Akpan AI. The neurological significance of abnormal natural killer cell activity in chronic toxigenic mold exposures. ScientificWorldJournal. 2003 Nov 13;3:1128-37. doi: 10.1100/tsw.2003.98. PMID: 14625399; PMCID: PMC5974762.
Schmidt S, Condorelli A, Koltze A, Lehrnbecher T. NK Cells and Their Role in Invasive Mold Infection. J Fungi (Basel). 2017 May 19;3(2):25. doi: 10.3390/jof3020025. PMID: 29371543; PMCID: PMC5715926.
Sandhu JK, Kulka M. Decoding Mast Cell-Microglia Communication in Neurodegenerative Diseases. Int J Mol Sci. 2021 Jan 22;22(3):1093. doi: 10.3390/ijms22031093. PMID: 33499208; PMCID: PMC7865982.
Hendriksen E, van Bergeijk D, Oosting RS, Redegeld FA. Mast cells in neuroinflammation and brain disorders. Neurosci Biobehav Rev. 2017 Aug;79:119-133. doi: 10.1016/j.neubiorev.2017.05.001. Epub 2017 May 10. Erratum in: Neurosci Biobehav Rev. 2017 Dec;83:774. doi: 10.1016/j.neubiorev.2017.10.030. PMID: 28499503.
Morey JN, Boggero IA, Scott AB, Segerstrom SC. Current Directions in Stress and Human Immune Function. Curr Opin Psychol. 2015 Oct 1;5:13-17. doi: 10.1016/j.copsyc.2015.03.007. PMID: 26086030; PMCID: PMC4465119.
Cohen, H., Kozlovsky, N., Matar, M. et al. The Characteristic Long-Term Upregulation of Hippocampal NF-κB Complex in PTSD-Like Behavioral Stress Response Is Normalized by High-Dose Corticosterone and Pyrrolidine Dithiocarbamate Administered Immediately after Exposure. Neuropsychopharmacol 36, 2286–2302 (2011). https://doi.org/10.1038/npp.2011.118
Si J, Yang J, Xue L, Yang C, Luo Y, Shi H, Lu L. Activation of NF-κB in basolateral amygdala is required for memory reconsolidation in auditory fear conditioning. PLoS One. 2012;7(9):e43973. doi: 10.1371/journal.pone.0043973. Epub 2012 Sep 5. PMID: 22957038; PMCID: PMC3434219.
Govindula, A., Ranadive, N., Nampoothiri, M. et al. Emphasizing the Crosstalk Between Inflammatory and Neural Signaling in Post-traumatic Stress Disorder (PTSD). J Neuroimmune Pharmacol 18, 248–266 (2023). https://doi.org/10.1007/s11481-023-10064-z
Exelby,G. Brainstem Hypoperfusion, Coat Hanger pain and Post-Exertional Malaise in POTS and Long COVID. 2024. https://www.mcmc-research.com/post/brainstem-hypoperfusion-coat-hanger-pain-and-post-exertional-malaise-in-pots-and-long-covid
Kempuraj D, Selvakumar GP, Thangavel R, Ahmed ME, Zaheer S, Raikwar SP, Iyer SS, Bhagavan SM, Beladakere-Ramaswamy S, Zaheer A. Mast Cell Activation in Brain Injury, Stress, and Post-traumatic Stress Disorder and Alzheimer's Disease Pathogenesis. Front Neurosci. 2017 Dec 12;11:703. doi: 10.3389/fnins.2017.00703. PMID: 29302258; PMCID: PMC5733004.
Kondashevskaya, M.V., Artemyeva, K.A. & Mikhaleva, L.M. The Synergistic Trio of Metabolic Master Regulators Sustaining a Vicious Circle of Pathological Processes in Post-Traumatic Stress Disorder. J Evol Biochem Phys 60, 988–1002 (2024). https://doi.org/10.1134/S0022093024030116
Harding CF, Pytte CL, Page KG, Ryberg KJ, Normand E, Remigio GJ, DeStefano RA, Morris DB, Voronina J, Lopez A, Stalbow LA, Williams EP, Abreu N. Mold inhalation causes innate immune activation, neural, cognitive and emotional dysfunction. Brain Behav Immun. 2020 Jul;87:218-228. doi: 10.1016/j.bbi.2019.11.006. Epub 2019 Nov 18. PMID: 31751617; PMCID: PMC7231651.
Kraft S, Buchenauer L, Polte T. Mold, Mycotoxins and a Dysregulated Immune System: A Combination of Concern? Int J Mol Sci. 2021 Nov 12;22(22):12269. doi: 10.3390/ijms222212269. PMID: 34830149; PMCID: PMC8619365.
Harcha PA, Garcés P, Arredondo C, Fernández G, Sáez JC, van Zundert B. Mast Cell and Astrocyte Hemichannels and Their Role in Alzheimer's Disease, ALS, and Harmful Stress Conditions. Int J Mol Sci. 2021 Feb 15;22(4):1924. doi: 10.3390/ijms22041924. PMID: 33672031; PMCID: PMC7919494.
Mojtaba Ehsanifar, Reihane Rajati, Akram Gholami, Joseph P Reiss. Mold and Mycotoxin Exposure and Brain Disorders. J. Integr. Neurosci. 2023, 22(6), 137. https://doi.org/10.31083/j.jin2206137
Biotoxins (indoor damp and mould) Clinical Pathway - Australian Government Department of Health and Aged Care. 2023.https://www.health.gov.au/resources/publications/biotoxins-indoor-damp-and-mould-clinical-pathway?language=en
Chai LY, Kullberg BJ, Vonk AG, Warris A, Cambi A, Latgé JP, Joosten LA, van der Meer JW, Netea MG. Modulation of Toll-like receptor 2 (TLR2) and TLR4 responses by Aspergillus fumigatus. Infect Immun. 2009 May;77(5):2184-92. doi: 10.1128/IAI.01455-08. Epub 2009 Feb 9. PMID: 19204090; PMCID: PMC2681752.
Comments